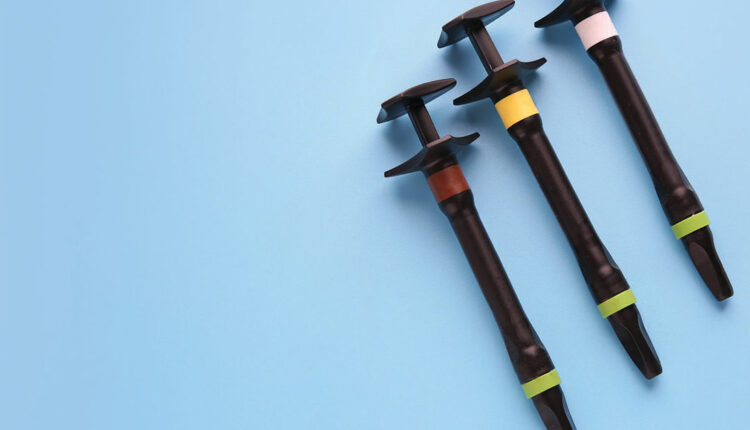
Biofilm Formation and Contact Angles on Dental Restorative Biomaterials
Understanding plaque formation on restorations is a crucial aspect of effective care.
The oral cavity is a complex biosphere inhabited by a diversity of microbial species. The oral microbiome exhibits a strong tendency to colonize hard and soft surfaces, including the teeth, tongue and mucosa — as well as any dental biomaterials used for restoration. This microbiome exists in the form of biofilms composed of bacterial colonies, surface substrates and fluid media. Biofilms are believed to be the primary cause of periodontal disease and caries (Figure 1A).1 While much has been written about biofilm and antibacterial materials in the field of medical devices,2–5 literature on dental biomaterials is more scarce. Yet that is beginning to change, and recent interest has focused on dental implants.6–8 Biofilm formation on biomaterials may degrade the material and roughen its surface (Figure 1B), thereby enhancing bacterial adhesion and initiating secondary caries and periodontal pathology. Thus, understanding biofilm formation on restorations is crucial to dental professionals.
Although various factors, such as surface roughness, surface energy and chemical composition, may affect biofilm formation, the influences that affect bacterial adhesion to restorative dental materials remain unclear. In this paper, we present a novel chemostat model using biomaterials substrates and multiple bacterial species as a means of investigating biofilm formation. A simple method of measuring contact angles to correlate the biofilm data is also presented.
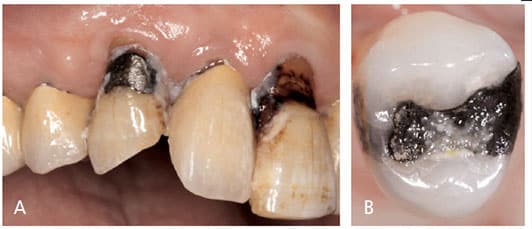
MATERIALS AND METHODS
Specimen Preparation: Eight readily available biomaterials were selected, including gold casting alloy, dental amalgam, zirconia, base-metal alloy, resin composite, glazed and ground porcelain, glass ionomer, and lithium disilicate glass-ceramic materials. Human dentin from extracted molars and Teflon material were used as positive and negative controls, respectively, making a total of 11 test groups. The generic name and components of the tested biomaterials are listed in Table 1. Disk-shaped specimens (with a 15-mm diameter and 3.0-mm thickness) were fabricated, with three specimens for each test material. Polishing of the surfaces metallurgically was performed in water with special rotary equipment using 1200-grit silicone-carbide paper. After polishing, the test specimens were washed with distilled water in an ultrasonic bath for 60 seconds and rinsed twice with distilled water before sterilization.
Chemostat Setup: The authors chose to cultivate the oral mixed culture in continuous culture at a known growth rate and physiological state, as suggested by Dezelic et al.9 A custom-built parallel flow chamber was used to investigate the bacterial attachment and biofilm formation on the various substrates. The mixed oral consortium was grown in a chemostat that forms one portion of a biofilm accumulation study flow system. The culture was continuously added at a constant reaction volume to ensure a well-mixed bioreactor (Figures 2A and 2B).10
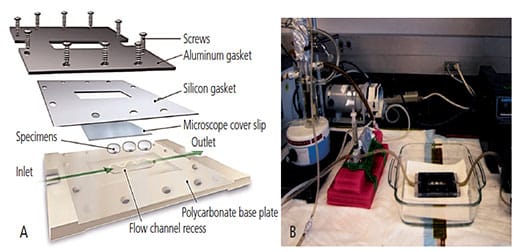
Once the mixed culture community reached a steady state in the chemostat, an additional supply line was used to pump reactor contents through a parallel-plate flow cell containing removable test resin sample plugs. The system was designed to control the bacterial culture challenge through the flow cell in order to ensure constant species distribution and overall growth activity.
Parallel-plate flow chambers were constructed of clear acrylic in two halves, with a flow channel machined into the inside face of one half. Sterilized specimens were placed flush with the interior surface of the flow channel. Using a variable-speed peristaltic pump downstream of the flow cells, a volumetric flow rate (1.0 ml/minute) was generated to simulate pertinent fluid shear conditions (approximately 2 N/m2).
Microbial Mixed Culture and Evaluation: A microbial mixed culture consisting of five oral species, Streptococcus mutans ATCC #700610, Streptococcus gordonii ATCC #49818, Actinomyces naeslundii ATCC #51655, Porphyromonas gingivalis ATCC #33277, and Fusobacterium nucleatum ATCC #25586, was continuously grown in the chemostat. The bacteria were analyzed fluorescently using conjugated antibodies and confocal laser scanning microscopy. Three-dimensional image analysis software was used to analyze and manipulate the images. The oral biofilm cell viability was monitored using a live-dead assay.
Wettability and Contact Angle Measurement: The wettability of biomaterials in vitro was evaluated by measuring the contact angle at the liquid-solid interface. We employed the sessile drop technique by depositing a uniform volume of distilled water on the surface of a solid, coupled with the standard arrangement for optical measurement of the contact angle using drop shape analysis. The static contact angle was recorded with photographic imaging within 5 seconds, and the resulting images were analyzed by computer. Three specimens from each group were measured on contralateral sides and data were analyzed with ANOVA and post hoc Tukey HSD test at α = 0.05.
RESULTS
Live and Dead Cells Measurement: Live and dead cell counts (Figure 3 and Figures 4A and 4B) after 48 hours revealed that gold casting alloy, amalgam and zirconia materials harbored low numbers of both live and dead cells. Base-metal alloy, resin composite and lithium disilicate glass-ceramic surfaces retained significantly higher numbers of dead cells compared to controls (dentin and Teflon). In terms of biofilm formation, glazed porcelain, glass ionomer, Teflon and human dentin experienced significantly higher bacterial growth.
Contact Angle Measurement: Surface wettability significantly influences biocompatibility response and is often calculated by measuring the material’s contact angle. Figures 5A and 5B illustrate images taken of typical sessile drop experiments for glazed porcelain (hydrophilic) and Teflon (hydrophobic), respectively. Our results show the materials tested present different contact angles, which can be explained by surface characteristics, such as composition, fillers and topography. It appears that Teflon as a negative control showed the highest values when measured with water (106° ± 3.6°), while dentin as a positive control yielded low contact angle values of 19° ± 4.1° (Figure 6).
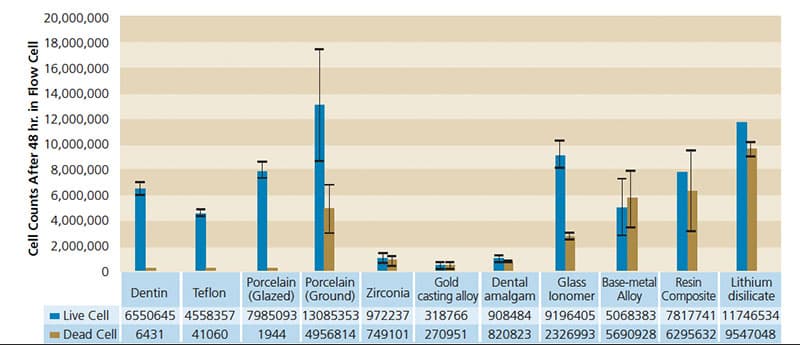
DISCUSSION
Cell viability is an important parameter in tissue engineering and culture studies to evaluate the effect of environmental conditions on cell behavior. One of the most salient observations resulting from our biofilm data was the difference between glazed and ground porcelain. We hypothesized that glazed porcelain would have less live cells than ground porcelain specimens. In general, under clinical conditions rough surfaces accumulate more biofilm than smooth surfaces. Our results support this hypothesis. It must be cautioned that although surface roughness may influence initial biofilm adherence, differences may vanish following growth and maturation.
Another observation involved biofilm formation on amalgam, gold casting alloy and zirconia surfaces. Although we hypothesized that metal-containing specimens, such as high noble gold and amalgam, will have a low live cell count, the zirconia group’s result may be a surprise to many practitioners. Zirconium dioxide, or zirconia, is a white densely crystalline oxide of zirconium. Zirconia actually is stabilized with yttria (yttrium oxide) so it can be used as a strong base material in some full-ceramic crown restorations. Yttrium has been shown to be dangerous to humans and animals. On the other hand, monolithic lithium disilicate is a glass ceramic that behaves similarly to ground porcelain and composite, as well as glass ionomer in terms of live cell counts.
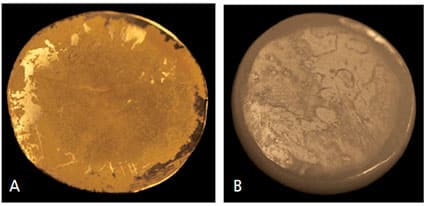
Dental amalgam and gold casting alloy are metallic materials with high electrical conductivity that plays a role in bacterial adhesion. This is because bacteria are negatively charged and attracted to positively charged conducting metallic materials. However, we did not observe that biofilm readily forms on amalgam and gold casting alloy. Auschill et al11 observed that biofilm on gold has low viability. However, in separate studies Wataha12 and Wataha et al13 observed that gold actually can be released into a fluid medium. This might explain the clinical longevity of amalgam and gold restorations, which may be more resistant to secondary caries formation than other materials.
Another commonly used base-metal alloy has a high dead cell count on the surface. The base-metal alloy is a nickel-chrome alloy that can be used for ceramo-metal restoration. Commonly, it is used for bonded bridges, cast dowel cores, inlay-retained bridges and anterior veneer bridges. One of the major shortcomings of nickel-chrome alloy, however, is its proven cytotoxicity, and its use has been gradually phased out.
An important observation is that glass ionomer has high live cell attachment. In this study, both porcelain and glass ionomer presented low contact angles (Figure 5A), indicating these materials have a tendency to be more hydrophilic than hydrophobic, which makes them more susceptible to biofilm attachment and formation. Roughness is also a significant factor. The positive flow pressure (2 N/m2) in the chemostat and constant flow of colonizing organisms can cause severe deterioration of the glass ionomer surface within 48 hours, which, in turn, promotes biofilm formation and more extensive deterioration. Porcelain may not be as affected by flow erosion, however.
Glass ionomer has been prescribed for patients at high caries risk due to its fluoride content. In this study, we did not observe that fluoride reduces the amount of bacterial growth and biofilm formation. This suggests that either fluoride is not a dominant factor in controlling biofilm, or the concentration was too low to be effective. The clinician must consider the balance between potential roughness and the protective effects of fluoride.
Resin composite has biofilm and contact angle data comparable to glass ionomer. It has been observed that resin composites cause more secondary caries than amalgam or glass ionomers. One cannot explain the difference based on our findings. There is another pathway whereby the components in resin composite materials can trigger other molecular events. Khalichi et al14 found that triethylene glycol, as the ether portion of triethylene glycol dimethacrylate, modulates the expression levels of glucosyltransferase B involved in biofilm formation. Clearly, such observations deserve more in-depth study.
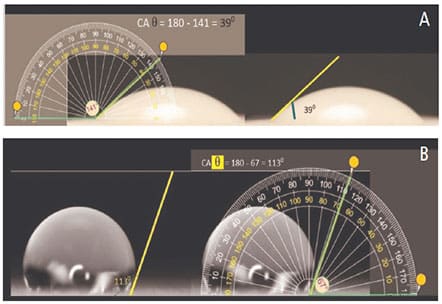
porcelain (A), and a hydrophobic surface: Teflon (B).
The contact angle measurement method is probably the most expeditious way to determine the hydrophobicity of material surfaces. The angle is very high for water if the substrate is hydrophobic, as shown in Figure 5B. Contact angles change with surface topography, surface tension of the liquid, surface energy of the substrate, and level of interaction between the liquid and solid. Our results show the materials tested presented different surface characteristics, such as composition, fillers, presence of fluoride particles, and topography. It appears that surface treatment with polishing materials can alter their wettability. All the materials analyzed showed contact angles ranging between our positive and negative controls (Figure 6). The results of this study are partially compatible with previous findings.15–17 Our results show that as a porous and hydrophilic material, dentin’s measured angle starts low and remains variable because the droplets quickly dissipate.
We tried to set a time frame within 5 seconds to compare fairly (Figure 5A). Although Teflon is inherently hydrophobic and cannot be wet easily, its behavior in terms of biofilm formation is unexpected. We observed that biofilm with live cells can absorb into the Teflon surface. This has clinical significance because waterlines in dental operatories are usually made of polymer tubing and other plastic materials that behave similarly to Teflon. Biofilms in dental unit water systems can become a biohazard if systems are not subject to periodic cleaning and maintenance, as per accepted asepsis protocols.18,19
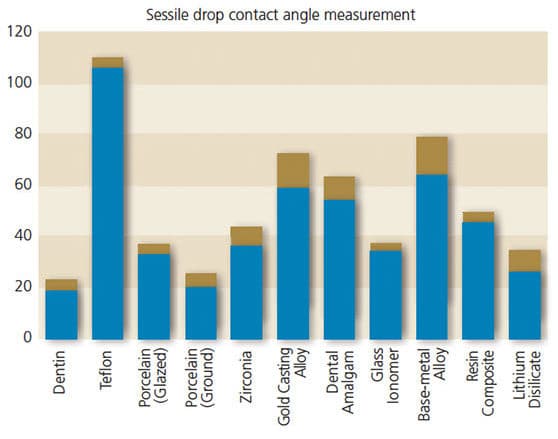
CONCLUSION
The limitations of this study lie in the small sample size and large variations of the biofilm data. In addition, we studied only five bacterial species, so caution is advised before extrapolating these findings to human cells and in vivo conditions. Among the limitations of the contact angle measurement data are the demands on a reasonably planar surface in order to obtain reproducible results. We attempted to control this variable by employing a standardized polishing protocol. Our research suggests that while roughness is a significant factor, other factors — such as surface charges, energy and chemical composition — may also play a role.
Within the limits of this study, we conclude the techniques described in this paper are feasible for studying biofilm formation on dental biomaterials, and comparable to other models.20 This may have ramifications for improving future materials and clinical technique, as understanding how biofilm forms on restorations is crucial to effective patient care.
KEY TAKEAWAYS
- Biofilm colonization of dental biomaterials may degrade the material and roughen its surface, thereby enhancing bacterial adhesion and initiating secondary caries and/or periodontal pathology.
- This paper presents a novel model consisting of biomaterial substrates and multiple bacterial species used to investigate biofilm formation. A simple method of measuring contact angles to correlate the biofilm data is also presented.
- Oral biofilm cell viability was monitored using a live-dead assay.
- Surface wettability significantly influences biocompatibility response and is often calculated by measuring the material’s contact angle.
- In general, rough surfaces harbor more biofilm than smooth surfaces; the results of this investigation support this hypothesis.
- Reporting in separate studies, Wataha12 and Wataha et al13 observed that gold actually can be released into a fluid medium. This might explain the clinical longevity of amalgam and gold restorations, which may be more resistant to secondary caries formation than other materials.
- The authors suggest the techniques described in this paper are feasible for studying biofilm on dental biomaterials, and may have ramifications for improving future materials and clinical technique.
REFERENCES
- Marsh PD. The role of microbiology in models of dental caries. Adv Dent Res.1995;9:244–254, discussion 255–269.
- Francolini I, Vuotto C, Piozzi A, Donelli G. Antifouling and antimicrobial biomaterials: an overview. APMIS. 2017;125:392–417.
- Veerachamy S, Yarlagadda T, Manivasagam G, Yarlagadda PK. Bacterial adherence and biofilm formation on medical implants: a review. Proc Inst Mech Eng H. 2014;228:1083–1099.
- Campoccia D, Montanaro L, Arciola CR. A review of the biomaterials technologies for infection-resistant surfaces. Biomaterials. 2013;34:8533–8554.
- Busscher HJ, Ploeg RJ, van der Mei HC. SnapShot: biofilms and biomaterials; mechanisms of medical device related infections. Biomaterials. 2009;30:4247–4248.
- Preethanath RS, AlNahas NW, Bin Huraib SM, et al. Microbiome of dental implants and its clinical aspect. Microb Pathog. 2017;106:20–24.
- Abdulkareem EH, Memarzadeh K, Allaker RP, Huang J, Pratten J, Spratt D. Anti-biofilm activity of zinc oxide and hydroxyapatite nanoparticles as dental implant coating materials. J Dent. 2015;43:1462–1469.
- Busscher HJ, Rinastiti M, Siswomihardjo W, van der Mei HC. Biofilm formation on dental restorative and implant materials. J Dent Res. 2010;89:657–665.
- Dezelic T, Guggenheim B, Schmidlin PR. Multi-species biofilm formation on dental materials and an adhesive patch. Oral Health Prev Dent. 2009;7:47–53.
- Marsh PD, Bradshaw DJ. Physiological approaches to the control of oral biofilms. Adv Dent Res. 1997;11:176–185.
- Auschill TM, Arweiler NB, Brecx M, Reich E, Sculean A, Netuschil L. The effect of dental restorative materials on dental biofilm. Eur J Oral Sci. 2002;110:48–53.
- Wataha JC. Biocompatibility of dental casting alloys: a review. J Prosthet Dent. 2000;83:223–234.
- Wataha JC, Craig RG, Hanks CT. The release of elements of dental casting alloys into cell-culture medium. J Dent Res. 1991;70:1014–1018.
- Khalichi P, Singh J, Cvitkovitch DG, Santerre JP. The influence of triethylene glycol derived from dental composite resins on the regulation of Streptococcus mutans gene expression. Biomaterials. 2009;30:452–459.
- Abi-Rached FO, Martins SB, Campos JA, Fonseca RG. Evaluation of roughness, wettability, and morphology of an yttria-stabilized tetragonal zirconia polycrystal ceramic after different airborne-particle abrasion protocols. J Prosthet Dent. 2014;112:1385–1391.
- Nakamura M, Hori N, Namba S, Toyama T, Nishimiya N, Yamashita K. Wettability and surface free energy of polarised ceramic biomaterials. Biomed Mater. 2015;10:011001.
- Spriano S, Sarath Chandra V, Cochis A, et al. How do wettability, zeta potential and hydroxylation degree affect the biological response of biomaterials? Mater Sci Eng C Mater Biol Appl. 2017;74:542–555.
- Wirthlin MR, Marshall GW Jr, Rowland RW. Formation and decontamination of biofilms in dental unit waterlines. J Periodontol. 2003;74:1595–1609.
- Walker JT, Bradshaw DJ, Finney M, et al. Microbiological evaluation of dental unit water systems in general dental practice in Europe. Eur J Oral Sci. 2004;112:412–418.
- Rudney JD, Chen R, Lenton P, et al. A reproducible oral microcosm biofilm model for testing dental materials. J Appl Microbiol. 2012;113:1540–1553.
Shuli Deng, DDS, MS, was supported by the Natural Science Foundation of Zhejiang Province and General Project of Health and Family Planning Commission of Zhejiang Province. The contributions of Daniel C. N. Chan, DMD, MS, DDS, were partially supported by the Washington Dental Service Endowment. Kwok-Hung Chung, DDS, PhD, has nothing to disclose.
From Decisions in Dentistry. September 2019;5(8):12—16.