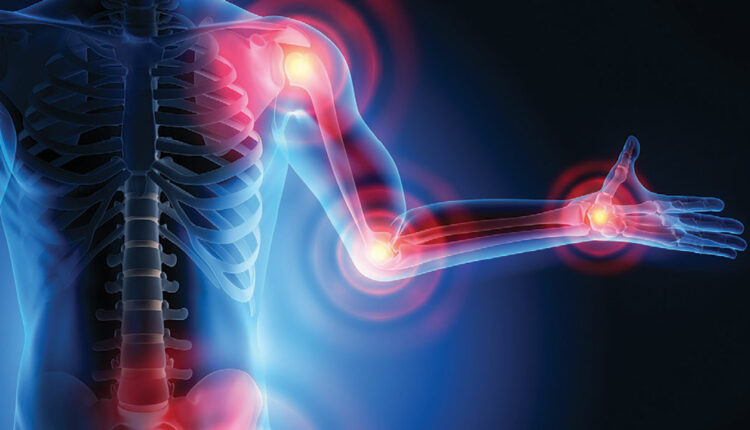
The Impact of Porphyromonas Gingivalis on Systemic Health
This oral bacterium implements strategies to invade the various tissues beyond the periodontium
This course was published in the June 2023 issue and expires June 2026. The authors have no commercial conflicts of interest to disclose. This 2 credit hour self-study activity is electronically mediated.
AGD Subject Code: 490
EDUCATIONAL OBJECTIVES
After reading this course, the participant should be able to:
- Identify how Porphyromonas gingivalis enters into systemic circulation.
- Discuss the relationship between P. gingivalis and cardiovascular disease, diabetes mellitus, and rheumatoid arthritis.
- Explain the role of oral health professionals in using the P. gingivalis-systemic disease association to help patients improve their overall health.
This article is Part 2 of the two-part series exploring the role of Porphyromonas gingivalis in systemic diseases. Part 1 examined the bacterium’s unique features and virulence factors and appeared in the February 2023 issue of Decisions in Dentistry.
Armed with the ability to transform its own environment and the entire periodontal microbiome as well as modify the host response, the influence of Porphyromonas gingivalis extends beyond the oral cavity.1–3 It is capable of invading distant tissues and organs and is involved in the pathogenesis of systemic diseases including cardiovascular disease (CVD), atherosclerosis, hypertension, myocarditis, and myocardial infarction (MI). This periodontal pathogen can also impact autoimmune diseases including rheumatoid arthritis (RA); neurodegenerative diseases such as Alzheimer disease; several cancers, including oral, esophageal, and pancreatic; and metabolic diseases such as diabetes mellitus.1,2 Moreover, ongoing research implicates P. gingivalis as a possible risk factor in psychological conditions such as depression; adverse pregnancy outcomes; and respiratory diseases such as pneumonia and chronic obstructive pulmonary disease.1 Research is ongoing to elucidate the degree to which this bacterium is involved in the etiology and pathogenesis of these and other systemic diseases.
Transient bacteremia — the presence of periodontal pathogens in the bloodstream following therapeutic dental procedures with manipulation of the gingival tissues and daily activities (such as toothbrushing, flossing, and chewing) — is a well-documented occurrence.4–7 Some patients may be at increased risk for bacteremia following dental procedures and self-care practices, depending on the degree of associated periodontal tissue manipulation and the density of pathogenic bacteria present. They may require antibiotic prophylaxis prior to dental care. Although P. gingivalis is not the bacterium implicated in the cardiac conditions that require antibiotic prophylaxis, it too is found in the bloodstream along with other periodontal pathogens following routine oral activities and therapeutic procedures.4,6
A systematic review of the literature evaluating the presence of bacteremia following various dental procedures found that oral bacteria, including P. gingivalis, were present in the bloodstream following periodontal probing (33.7%) and nonsurgical periodontal therapies, including hand and ultrasonic scaling (46%).5 Although the duration and magnitude of the resulting bacteremia could not be determined, it is estimated that multiple, repeated exposures due to daily activities, such as brushing and chewing — especially in patients with periodontitis — can result in ~3 hours of daily bacteremia.4 This invasion of P. gingivalis in the bloodstream can facilitate its distribution to distant sites and infection of nonperiodontal cells/tissues, including epithelial, endothelial, smooth muscle cells, placental cells, and neurons.1,3,4,8
Remarkably, just like it relies on the microbiome and its pathobionts for survival and nutritional needs, P. gingivalis also benefits from other biofilm bacteria in its invasion of host tissues.9,10 For example, Fusobacterium nucleatum co-infection increases P. gingivalis’ invasion of the gingival epithelial and aortic endothelial cells by 2 times to 20 times in vitro.11 This confirms the polymicrobial nature of periodontal infection and the role of P. gingivalis as a key pathogen.3,9,10
The invasion mechanisms of host cells by P. gingivalis differ based on its strain and the presence of virulence factors, such as fimbriae and outer membrane vesicles (OMVs), as well as the host cell/tissue type.4 Nevertheless, the invasion process generally presents in five stages (Figure 1) and it demonstrates the bacterium’s supreme adaptive skills. Notably, upon its exit from the infected host cell, P. gingivalis can infect neighboring cells of the same or different type, and although cell-to-cell contact enhances transmission, it is not absolutely necessary as P. gingivalis can exit into the intercellular environment and infect new cells from the outside. The bacterium can also become dormant and remain intracellularly undetected by the host’s immune system and unreachable by antibiotics.4,12
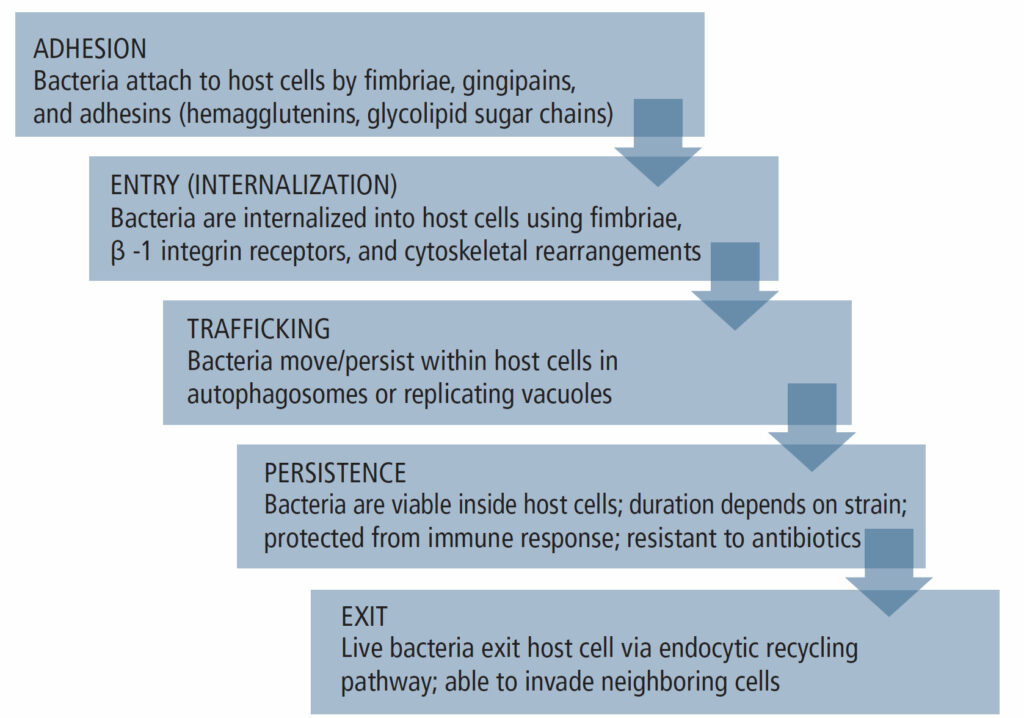
CARDIOVASCULAR DISEASE
Extensive research has identified the specific role of P. gingivalis in systemic inflammation, particularly CVD.12 Specifically, the role of P. gingivalis in the development of abdominal aortic aneurysms, a permanent localized widening of the abdominal aorta, has been studied. Additionally, the periodontal pathogen may play a role in atherosclerotic CVD, characterized by the progressive accumulation of lipids, calcium, cells, and other components on the surface of the vascular wall, resulting in coronary artery disease that may lead to MI and ischemic stroke.1,2 Recently, the link between hypertension and periodontal diseases has been revealed, and the specific etiologic/pathogenic mechanisms of P. gingivalis virulence factors and live bacteria are being actively investigated.
The presence of periodontal pathogens has been associated with aneurismal disease by promoting the proliferation of aortic smooth muscle cells — increasing oxidative stress, inflammation, and proteolysis.1,2,12 In fact, P. gingivalis was detected in aneurismal specimens at the highest rate (85%).1 P. gingivalis is also among the most common microorganisms found in atherosclerotic plaques, and it promotes endothelial dysfunction and increases vascular permeability, resulting in edema via multiple mechanisms and virulence factors, including its fimbriae (FimA) and OMVs.1,2,13 OMVs continuously released by P. gingivalis are particularly important in the systemic dissemination. As nanomolecules (50 to 250 nm), they have better access into the deep tissues where live bacteria cannot reach, and their endovascular effects are concentration-dependent.2 The lipopolysaccharides of P. gingivalis promote the accumulation of lipoproteins in macrophages and can stimulate the proliferation and calcification of the vascular smooth muscle cells. Additionally, P. gingivalis has a procoagulant effect in endothelial cells. It promotes platelets’ activation and aggregation, thus impairing vasomotor function and further fostering atherosclerotic plaque progression and instability.1,2,7 Repeated in vitro exposures of coronary artery endothelial cells — both lipopolysaccharides and live P. gingivalis — were shown to stimulate angiotensin-II production, induce vasoconstriction, and play a role in the onset and progression of hypertension.7
MI is associated with chronic inflammation, including periodontitis, and altered immune response. A recent meta-analysis that evaluated the detection of periodontal pathogens in the atherosclerotic plaques of patients with MI, found P. gingivalis in ~40% of samples, followed by Aggregibacter actinomycetemcomitans.14 Producing endotoxins and various virulence factors, periodontal bacteria within the atherosclerotic plaque interact with the endothelial cells, affecting the immune response and promoting the prothrombotic reaction.1,14 In animal studies, P. gingivalis was shown to induce myocarditis and MI. It promotes cardiomyocyte apoptosis (programmed death) by stimulating an oxidative stress occurrence, which also prevents the healing process of the infarcted myocardium.1
Systemic inflammation linked with CVD and atherosclerotic plaque formation correlates with the severity of periodontal diseases. Further supporting this oral-systemic relationship is the reduction in systemic and endovascular inflammation following nonsurgical periodontal therapy. This is evidenced by improved endothelial function and a reduction in inflammatory biomarkers.1,12
DIABETES MELLITUS
Periodontal diseases became known as the sixth comorbidity associated with diabetes, after studies revealed periodontal diseases are disproportionately more prevalent in patients with Type 1 and Type 2 diabetes and pre-diabetes.2,15,16 Novel findings of P. gingivalis’ virulence factors and the bacterium’s impact on metabolic homeostasis have led to a better understanding of the bidirectional relationship between the two diseases.2,15 Additional studies have revealed periodontal diseases as a contributing factor in the development of diabetes.1,17 Conversely, diabetes is considered a risk factor for the progression of periodontal diseases. A recent systematic review quantified that Type 2 diabetes increased the risk of developing periodontitis by 34%, whereas severe periodontitis raised the potential of acquiring Type 2 diabetes by 53%.15
The inflammatory process of the periodontium and the host system is the most studied pathway linking periodontal diseases and diabetes. Both diseases undergo inflammatory pathogenesis and progression, and initiate inflammatory responses that disrupt distant organ homeostasis.16 The keystone pathogen of periodontal diseases — P. gingivalis — increases systemic inflammation via endotoxemia, translocation, and altered gut microbiome, or by causing a dysfunction of the host immune response.1,2,13,15,16 The fimbriae of P. gingivalis, specifically, FimA, have demonstrated motility capabilities for disseminating into neighboring and distant cells.10 Although intervention studies on nonsurgical periodontal therapy in patients with diabetes revealed reduced glycated hemoglobin (HbA1C), a recent longitudinal study on patients with diabetes and periodontal disease with P. gingivalis-FimA in the periodontal pockets showed an increase in HbA1C, implying a relationship between P. gingivalis virulence and glucose control in diabetes. 15 However, the exact mechanisms of P. gingivalis-FimA and its correlation to glucose control are still under investigation.
Gingipains, produced by P. gingivalis, enter the bloodstream through the vascular endothelial cells within the periodontal pocket, and can induce insulin resistance by inhibiting glucose storage (glycogenesis) and boosting hepatic gluconeogenesis, which is a major contributor to high blood sugar.1,2,15,16 Animal and human pancreatic sample analyses have highlighted gingipains’ role as one of the chief virulence factors of P. gingivalis in the pathogenesis of diabetes.1,2,13 Translocated gingipains were shown to concentrate mainly within or closely near the nuclear regions of pancreatic beta cells, which produce and release insulin in response to fluctuations of blood glucose levels. Researchers suggest gingipains may cause premature cell changes within the nuclei of pancreatic beta cells, resulting in altered normal insulin production.1,2,13,15 The virulence of OMVs is also of particular interest in the connection between periodontal diseases and diabetes, as they are thought to be the driving force behind P. gingivalis translocation.2,15 P. gingivalis’ OMVs are more virulent and better at infiltrating deeper tissues, depending on the environmental make-up of lipids, proteins, and nucleic acids. OMVs can transport active gingipains to the liver, altering glucose metabolism; this is crucial since hepatic glucose production accounts for 90% of total glucose synthesis in the body.2,18,19
Diabetes, on the other hand, contributes to the pathogenesis of periodontal diseases by increasing the body’s inflammatory response and shifting the oral microbiome into dysbiosis. This occurs as inadequate management of blood glucose levels can increase the concentration of systemic inflammatory markers within the gingival crevicular fluid (GCF).15,20 Moreover, protein byproducts left over from diabetes metabolic pathology provide P. gingivalis the fuel needed to secure the extra heme required to maintain a persistent infection.10 In a study elucidating a novel theory in the bidirectional connection between diabetes and periodontal diseases, the term “trained immunity” was coined to describe the capacity of the body’s adaptive immune system to store immunological memory to provide continuous and quick protection against foreign pathogens.15 It’s been suggested that the release of periodontal bacteria and inflammatory cytokines from the oral microbiome, coupled with diabetes-induced hyperglycemia, may stimulate changes in the epigenetic coding of dendritic cells and monocytes (peripheral myeloid cells) and bone-marrow precursors (phagocytic cells). This may stimulate the overactivity of myeloid cells, which can then react more quickly to a second inflammatory encounter even if it is only perceived.15
RHEUMATOID ARTHRITIS
Brandtzaeg and Kraus20 uncovered findings of anti-collagen antibodies in infected periodontal sites, which provided insight into the connection between autoimmune reactions and periodontal diseases. This contributed to current understanding that alveolar bone destruction involves the formation of systemic immune complexes, and the progression of periodontal diseases occurs from a prolonged dysbiosis of the oral microbiota maintained by inflammophilic microbes, causing a continued disrupted function and deficiency in the regulation of inflammatory responses.10,20 Although the underlying pathogenic mechanisms of autoimmune diseases, such as RA, are continually being explored due to their complex etiologies, the persistent oral dysbiosis of periodontal diseases is considered a contributing factor.20,21
RA is characterized by inflammation of the synovial membranes that leads to the breakdown of cartilage and bone due to an overreactive immune response of anticitrullinated protein antibodies (ACPAs), the most sensitive and specific biomarker identified in RA cases.20–23 Studies have shown a higher prevalence of periodontal diseases among patients with RA and suggest a connection and plausible bidirectional relationship.20,22 These diseases share strong similarities in immunological, biological, and genetic traits, exhibiting a unique amassing of cytokines, oxygen radicals, and prostaglandins within their respective inflamed sites. Patients with RA and periodontitis were found to have higher percentages of neutrophils in their synovial fluid (80% to 90%) and GCF (80% to 95%).22 Researchers attribute the connection between periodontal diseases and RA to P. gingivalis.
P. gingivalis’ unique contribution to the pathogenesis of RA is supported by the fact that it is the only human bacterium able to produce peptidyl arginine deiminase. This enables the conversion of arginine residues into citrulline.20–22 Chronic periodontal inflammation high in citrulline proteins may lead to an immune-stimulating pathway that increases humoral immune response and an unrelenting production of ACPAs, thus impacting immunological tolerance and inciting autoimmunity.23 Although citrullination and ACPAs are minimally present in healthy periodontal tissues, chronic periodontal infection causes a repeated release and high degree of ACPAs found within the GCF of patients with periodontal diseases.20 Likewise, peptidyl arginine deiminase in the joints produces an excess of citrullinated proteins, which are then bound in large numbers by the ACPAs, consequently releasing pro-inflammatory cytokines responsible for breaking down of joint structures, a distinctive feature of RA.22,23
Autoimmunity and immunodeficiencies were traditionally thought to have independent activation pathways. However, it is now understood that dysfunction of immune signaling and regulation are common threads shared by both immunity disorders,20,22 and several of the same inflammatory pathways as periodontal diseases.
P. gingivalis can limit the complement cascade and restrain leukocyte death by activating and exploiting the C5a-receptor signaling pathway.20,22 C5a complement peptide is an essential modulator of the inflammatory response present in alveolar and synovial tissue, highlighting the corresponding pathophysiology of RA and periodontitis. Another viable conduit in systemic dysbiosis and immune dysfunction under investigation is the translocation of P. gingivalis with its microbiome-altering abilities and its coaggregation pathobiont actions found in the gut flora.20,22 Studies on murine (mouse) models that introduced P. gingivalis into the gut demonstrated an altered intestinal microflora and increased manifestation or aggravation of arthritis, including an increase in the production of proinflammatory cytokines observed at both the intestinal and extra-intestinal levels.20,21
CONCLUSION
Oral health professionals are at the forefront of preventing and treating not only dental and periodontal diseases, but also a growing list of systemic conditions associated with periodontal pathogens such as P. gingivalis. The oral-systemic connection, as well as the bi-directional nature of the many associations, offers dental professionals a unique and important opportunity to positively influence patients’ overall health.
REFERENCES
- Mei F, Xie M, Huang X, et al. Porphyromonas gingivalis and its systemic impact: current status. Pathogens. 2020;9:944.
- Zhang Z, Liu D, Liu S, Zhang S, Pan Y. The role of Porphyromonas gingivalis outer membrane vesicles in periodontal disease and related systemic diseases. Front Cell Infect Microbiol. 2021;10:585917.
- Hajishengallis G. Periodontitis: from microbial immune subversion to systemic inflammation. Nat Rev Immunol. 2015;15:30.
- Olsen I, Progulske-Fox A. Invasion of Porphyromonas gingivalis strains into vascular cells and tissue. J Oral Microbiol. 2015;7:28788.
- Horliana ACRT, Chambrone L, Foz AM, et al. Dissemination of periodontal pathogens in the bloodstream after periodontal procedures: a systematic review. PLOS ONE. 2014;9:e98271.
- Nishimura RA, Otto CM, Bonow RO, et al. 2017 AHA/ACC focused update of the 2014 AHA/ACC guideline for the management of patients with valvular heart disease: A report of the American College of Cardiology/American Heart Association Task Force on clinical practice guidelines. Circulation. 2017;135:e1159—e1195.
- Viafara-García SM, Morantes SJ, Chacon-Quintero Y, Castillo DM, Lafaurie GI, Buitrago DM. Repeated Porphyromonas gingivalis W83 exposure leads to release pro-inflammatory cytokynes and angiotensin II in coronary artery endothelial cells. Sci Rep. 2019;9:19379.
- Haditsch U, Roth T, Rodriguez L, et al. Alzheimer’s disease-like neurodegeneration in Porphyromonas gingivalis-infected neurons with persistent expression of active gingipains. J Alzheimers Dis. 2020;75:1361–1376.
- Hajishengallis G, Liang S, Payne MA, et al. A low-abundance biofilm species orchestrates inflammatory periodontal disease through the commensal microbiota and the complement pathway. Cell Host Microbe. 2011;10:497–506.
- Chopra A, Bhat SG, Sivaraman K. Porphyromonas gingivalis adopts intricate and unique molecular mechanisms to survive and persist within the host: a critical update. J Oral Microbiol. 2020;12:1801090.
- Saito A, Inagaki S, Kimizuka R, et al. Fusobacterium nucleatum enhances invasion of human gingival epithelial and aortic endothelial cells by Porphyromonas gingivalis. FEMS Immunol Med Microbiol. 2008;54:349–355.
- Mulhall H, Huck O, Amar S. Porphyromonas gingivalis, a long-range pathogen: systemic impact and therapeutic implications. Microorganisms. 2020;8:869.
- Okamura H, Hirota K, Yoshida K, et al. Outer membrane vesicles of Porphyromonas gingivalis: novel communication tool and strategy. Jpn Dent Sci Rev. 2021;57:138–146.
- Joshi C, Bapat R, Anderson W, Dawson D, Hijazi K, Cherukara G. Detection of periodontal microorganisms in coronary atheromatous plaque specimens of myocardial infarction patients: a systematic review and meta-analysis. Trends Cardiovasc Med. 2021;31:69–82.
- Barutta F, Bellini S, Durazzo M, Gruden G. Novel insight into the mechanisms of the bidirectional relationship between diabetes and periodontitis. Biomedicines. 2022;10:178.
- Polak D, Sanui T, Nishimura F, Shapira L. Diabetes as a risk factor for periodontal disease-plausible mechanisms. Periodontol 2000. 2020;83:46–58.
- Ohtsu A, Takeuchi Y, Katagiri S, et al. Influence of Porphyromonas gingivalis in gut microbiota of streptozotocin-induced diabetic mice. Oral Dis. 2019;25:868–880.
- Nara PL, Sindelar D, Penn MS, Potempa J, Griffin WST. Porphyromonas gingivalis outer membrane vesicles as the major driver of and explanation for neuropathogenesis, the cholinergic hypothesis, iron dyshomeostasis, and salivary lactoferrin in alzheimer’s disease. J Alzheimers Dis JAD. 2021;82:1417–1450.
- Petersen MC, Vatner DF, Shulman GI. Regulation of hepatic glucose metabolism in health and disease. Nat Rev Endocrinol. 2017;13:572–587.
- Suárez LJ, Garzón H, Arboleda S, Rodríguez A. Oral dysbiosis and autoimmunity: From local periodontal responses to an imbalanced systemic immunity. A review. Front Immunol. 2020;11:591255.
- Montgomery AB, Kopec J, Shrestha L, et al. Crystal structure of Porphyromonas gingivalis peptidylarginine deiminase: implications for autoimmunity in rheumatoid arthritis. Ann Rheum Dis. 2016;75:1255–1261.
- Koziel J, Potempa J. Pros and cons of causative association between periodontitis and rheumatoid arthritis. Periodontol 2000. 2022;89:83-98.
- Bereta G, Goulas T, MadeJ M, et al. Structure, function, and inhibition of a genomic/clinical variant of Porphyromonas gingivalis peptidylarginine deiminase. Protein Sci. 2019;28:478–486.
From Decisions in Dentistry. June 2023;9(6):42-45.