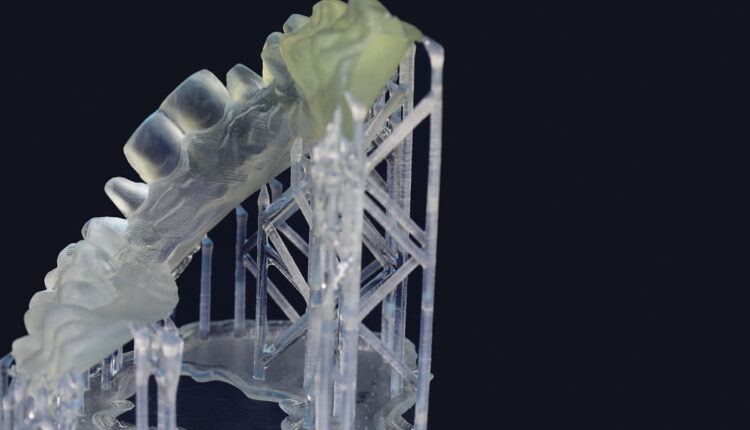
Stereolithography: The Future of Dental Fabrication
The advent of three-dimensional printing technology adds a new tool for the creation of dental appliances and prosthetics.
Increasingly popular with laboratories and practices, computer aided design/computer aided manufacturing (CAD/CAM) facilitates the design and fabrication of dental prosthetics and appliances. Clinicians using CAD/CAM technology experience improved accuracy and a reduced time in completing treatment because they are able to complete the work without the delays associated with traditional analog methods. In addition to chairside CAM milling equipment, the next generation of technology for designing and manufacturing onsite is available in the form of three-dimensional (3D) printers. Originally a very costly investment (upwards of $100,000), 3D printing is now a more viable option with the emergence of desktop printers. Desktop 3D printers now rival the quality of the more expensive units, but at a fraction of the cost — ranging from $4000 to $20,000 for clinical-grade accuracy and clinical-grade-approved materials. A range of materials is available for 3D printing, including dental resins, which may be used in the fabrication of temporary prosthetics, surgical guides, orthodontic models, retainers and aligners. Advances in dental resins for printing applications include transparency for optical clarity, flexible resins for patient comfort, and strong, high-impact resins that resist wear and fracture in oral applications.
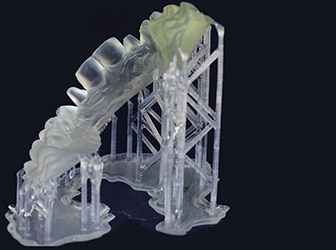
from a digital scan will be used in the fabrication of a
dental prosthesis.
The types of 3D printers commonly utilized in dentistry are stereolithography (SLA) and fused deposition modeling (FDM). When an item is printed using an SLA 3D printer, thin layers upon layers of resin are built up to create the computer-designed shape (Figure 1 and Figure 2). The liquid resin is contained in a tank, and either light-emitting diode or laser energy is used to form individual layers that can be stacked to create objects. The light causes the molecules to link in a process known as photopolymerization. By comparison, FDM printers use a similar additive approach of building layers, but FDM printing uses plastic filament instead of liquid resin. The plastic filament is fed through an extruder and melted, so that it may be deposited layer by layer into the print area in order to build the item.
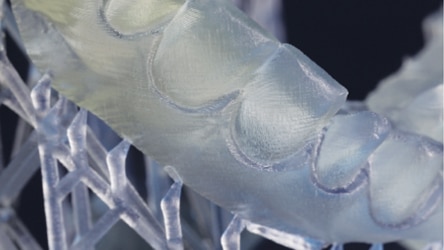
stereolithography technology.
Clinically, FDM printers are noteworthy for their comparatively simple workflow, as the item requires fewer finishing steps before it can be placed in the patient’s mouth. That noted, SLA printers achieve the most accurate results, and, for this reason, SLA technology will be the focus of this discussion. A great deal of the SLA printer’s accuracy can be attributed to the precision of the layers it creates (Figure 2). The thickness of the printed layers can be carefully controlled in what is known as adaptive slicing. If the surface has been designed with a high curvature, for example, thinner layers will be applied than would be the case for a low-curvature surface. Adaptive slicing yields a better surface quality than uniform slicing, as the staircase effect decreases, which minimizes variations in the cusp height across the layers.1 Although the completed piece has the smooth finish of a final product, 3D-printed items — such as surgical guides — require rigorous testing to ensure the production truly meets clinical standards.
QUALITY CONTROL
Before testing the accuracy of a 3D-printed item, the level of accuracy considered appropriate for clinical use must be established. The accuracy of an appliance (e.g., a surgical guide) can be tested by comparing the original design of the guide to the actual placement of the implant in the patient’s mouth. In clinical dentistry, many replicas of a patient’s dentition and oral tissues are needed in order to complete the case. If a desktop 3D printer could be capable of creating a level of clinical accuracy, it would certainly introduce an efficient and cost-effective means of creating dental models.
A study by Scherer2 notes that it is commonly asserted by clinicians that fixed restorations, such as crowns, should fit with marginal adaptation discrepancies within 10 to 30 μm — however, clinicians will accept a larger discrepancy in practice. Due to a range of factors — from the varying angulation of the radiographs taken to the sharpness of the clinician’s instruments — Scherer2 discovered that fit discrepancy is considered acceptable between 50 and 200 μm. That said, the average clinician would consider 100 μm to be the maximum acceptable discrepancy for a restoration to “fit.”
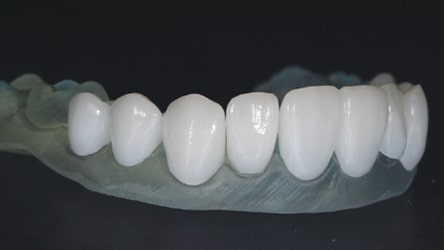
model ready for fit check and laboratory adjustment.
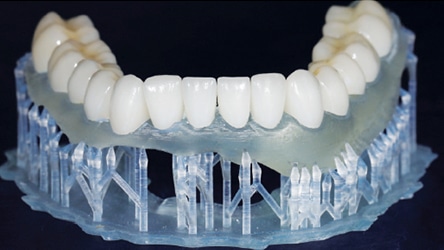
model; note the precision of the restorations produced using computer aided design/computer aided manufacturing technology.
Marginal adaptation is a key factor when defining the accuracy of a restoration. A marginal gap that is too large could lead to caries and premature loss of the restoration. Scherer continued to test the accuracy of dental models fabricated using a 3D printer, and found that printing at a 50-μm or 25-μm setting creates models that are considered acceptable by clinical standards. With the 25-μm setting, a higher level of accuracy is achieved because the layers are thinner. This results in a smoother and more detailed surface because the “stepping effect” is reduced. Scherer’s findings are validated by a study by Donovan and Chee,3 as, according to American Dental Association Specification 19, elastomeric impression materials used to fabricate precision castings must be able to reproduce fine detail of 25 μm or less.3 If 25 μm is the desired accuracy for impressions in order to clearly show the details of a patient’s case, a 3D printer capable of achieving this same accuracy can therefore be considered to be a suitable alternative for the fabrication of dental appliances (Figure 3 and Figure 4).
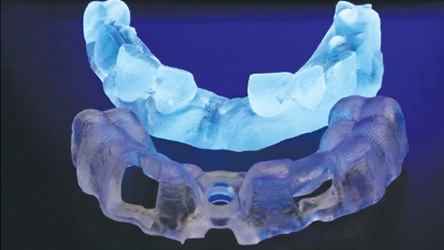
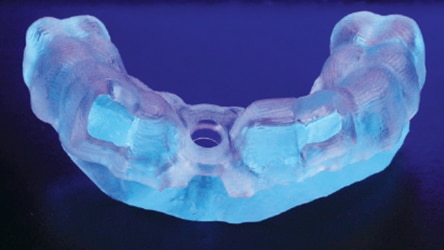
Surgical guides must be produced with exacting dimensional tolerances.4 A surgical guide may be created using a digital workflow in order to evaluate if the accuracy of the 3D printer meets acceptable clinical parameters. First, a cone beam computed tomography (CBCT) scan of the patient must be obtained before it can be examined using implant treatment planning software. Once treatment is planned virtually, the file is converted into an .STL (for STereoLithography) format and exported from the treatment planning software. The file may then be imported into the 3D printing software to begin the design and production of the guide (Figure 5, Figure 6 and Figure 7). In their study, “Digital Implantology with Desktop 3D Printing,” Whitley and Bencharit4 produced a surgical guide to be used in implant surgery. By comparing the presurgical plan to the actual implant placement in the patient, the authors were able to evaluate the accuracy of the 3D-printed guide. Images of the plan and implant placement were superimposed over one another, revealing an extremely accurate placement and thereby confirming the quality of a 3D-printed guide is acceptable for clinical dentistry.
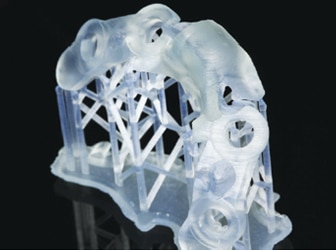
Technology is always improving on previous models, and this is certainly true for 3D printers. When 3D printing accuracy was tested in 2005 by Di Giacomo et al,5 researchers found that it was possible for a clinician to create a surgical guide using a 3D printer, however, it was also noted the technique needed improvement to provide better stability of the guide during surgery. These previous shortcomings have since been overcome. For example, improvements have been made to the accuracy of the 3D-printed guide, as later studies have proven 3D-printed dental appliances, such as implant guides, to be appropriate for clinical use.4
CLOSING THOUGHTS
Using a 3D printer in practice has helped the authors’ team overcome logistical challenges and improved clinical workflows by reducing the number of appointments a patient needs, all while increasing accuracy. Having access to other 3D technologies, such as digital intraoral scanning and CBCT imaging, aids in the acquisition of digital records that assist diagnosis and treatment planning. Using CAD/CAM software allows the office to design intricate dentistry, and put the plan into action through in-office printing technology. This provides the ability to rapid prototype models, both dental casts and medical bone models, such as an entire mandible or maxilla. Furthermore, it allows the creation of guides to help direct treatment, as well as surgical guides that increase the accuracy of implant placement. Having a 3D printer in a clinical setting also allows the testing of prosthetics, such as wax-ups and provisionals, prior to commencing final treatment.
KEY TAKEAWAYS
- The future holds unlimited possibilities for 3D printing. With increasing accuracy and quality of the finished piece — and an ever-expanding list of materials to use for printing — the potential for wider application in clinical dentistry is exciting.
- Computer aided design/computer aided manufacturing (CAD/CAM) facilitates the in-office design and fabrication of dental prosthetics and appliances.
- In addition to chairside CAM milling equipment, the next generation of technology for designing and manufacturing onsite is available in the form of three-dimensional (3D) printers.
- The use of dental resins and 3D printing allows in-office fabrication of temporary prosthetics, surgical guides, orthodontic models, retainers and aligners.
- Adjusting 3D printers to a 50-μm or 25-μm setting allows the creation of models that are considered acceptable by clinical standards. And at the 25-μm setting, a higher level of accuracy is achieved because the layers are thinner.
- With increasing accuracy and an ever-expanding list of materials to use for 3D printing, the potential for wider application in clinical dentistry is significant.
REFERENCES
- Bártolo PB. Stereolithographic Processes. In: Bártolo PB, ed. Stereolithography: Materials, Processes and Applications. New York, NY: Springer Science+Business Media, LLC; 2011:1–35.
- Scherer MD. Digital Dental Model Production with High Accuracy 3D Printing. Formlabs White Paper. Somerville, MA: Formlabs Inc; 2017:1–17.
- Donovan TE, Chee WL. A review of contemporary impression materials and techniques. Dent Clin North Am. 2004;48;2:445–470.
- Whitley D, Bencharit S. Digital Implantology with Desktop 3D Printing. Formlabs White Paper. Somerville, MA: Formlabs Inc; 2015:1–15.
- Di Giacomo GA, Cury PR, de Araujo NS, Sendyk WR, Sendyk CL. Clinical application of stereolithographic surgical guides for implant placement: preliminary results. J Periodontol. 2005;76:503–507.
The authors have no commercial conflicts of interest to disclose.
From Decisions in Dentistry. November 2017;3(11):18,21–22.