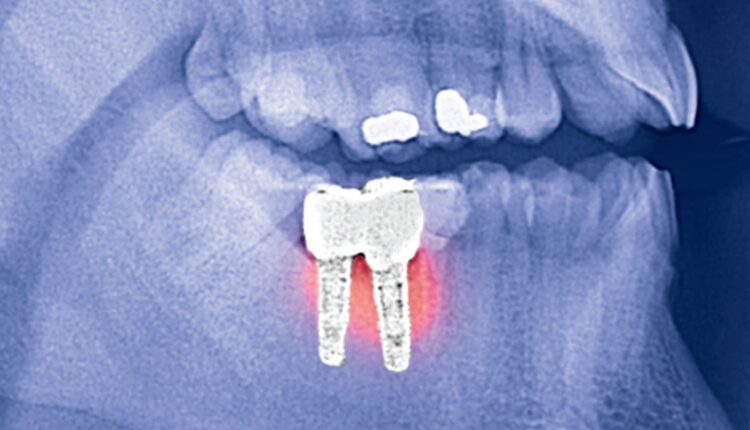
Use of Metal-titanate Nanoparticles to Combat Peri-implantitis
An exploration of a novel antimicrobial in the management of peri-implantitis.
This course was published in the October 2018 issue and expires October 2021. The authors have no commercial conflicts of interest to disclose. This 2 credit hour self-study activity is electronically mediated.
EDUCATONAL OBJECTIVES
After reading this course, the participant should be able to:
- Discuss the prevalence of dental implants and peri-implantitis.
- Explain the novel use of metal-based nanoparticles as antibacterials in dental implant therapy.
- Describe the properties of metal-based nanoparticles that show promise for use in managing peri-implant disease.
Given their high success rates and patient satisfaction compared to other treatments, dental implants are considered a viable solution for replacing missing teeth.1 According to the American Academy of Implant Dentistry, 3 million Americans have dental implants, and this number is growing by half a million per year.2 As far back as 2002, approximately 450,000 implants were being placed annually, with an expected 95% success rate and minimum complications.3 This reported success rate may be an overestimation, however, as numerous implants are lost due to peri-implantitis (bacterial infection), even after years of successful osseointegration.4
The inaugural classifications for peri‐implant mucositis and peri‐implantitis were recently developed.5,6 Peri‐implant mucositis is characterized by bleeding on probing and visual signs of inflammation. Peri‐implantitis is defined as a plaque–associated pathologic condition occurring in the tissue around dental implants that is characterized by inflammation in the peri‐implant mucosa and subsequent progressive loss of supporting bone (Figures 1A through 1C). Contrary to earlier studies that reported 5% to 8% prevalence of peri-implantitis,7 a more recent study by Konstantinidis et al8 suggests a 13% prevalence. Data from the University of Washington (UW) dental clinics indicate that approximately 25% of patients with dental implants develop peri-implantitis within 11 years following implant placement.9,10 (It must be cautioned that prior to 2018, no standardized definition or criteria existed for peri-implantitis, which may explain why previously reported percentages varied widely.)
While the routine use of antibiotics in peri-implantitis treatment is widespread, concerns about the overuse of antibiotics and antibiotic resistance have led to alternative therapies. As one example, nanoparticles are increasingly being used to target pathogens, and among the most recent additions to the growing list of metal-based antibacterials are gold titanate nanoparticles. This paper will explore the use of metal titanate nanoparticles and stripes/banding in the management of peri-implantitis.
Conventional treatment consists a nonsurgical phase, which includes debridement by mechanical means, ultrasonics or lasers (either alone or combined with antiseptic and/or antibiotic agents), and a surgical phase, utilizing either resective or regenerative techniques.11 Although it is not the purpose of this article to discuss the range of treatments for peri-implantitis, there are excellent systematic reviews on this topic and readers are encouraged to follow up.12,13
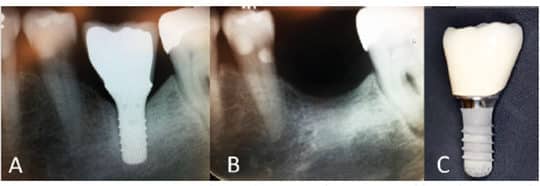
LOCAL AND SYSTEMIC DELIVERY OF ANTIMICROBIALS
The adjunctive use of systemic antimicrobials for periodontal therapy has been discussed for decades. In the absence of other infections, patients presenting with localized peri-implant conditions may be candidates for treatment by local drug-delivery devices. Local application of antibiotics by the insertion of tetracycline fibers for 10 days can provide a sustained high dose of this antibiotic into the affected site. Clinicians may suggest minocycline microspheres as an adjunct to mechanical therapy for managing peri-implant lesions, but this treatment may need to be repeated.14 As an alternative, providers may recommend a doxycycline gel that conforms to the contours of gingival surfaces and solidifies over them. Over several days, this gel gradually releases the antibiotic medication.
If the problem is generalized, specific microbiological information is collected and antibiotics are administered systemically. If peri-implantitis is associated with persistent periodontal disease, both conditions must be treated. To date, only one clinical trial (NCT02185209, recruiting) is actively investigating systemic administration of antibiotics for treating peri-implantitis.9 Oftentimes, the pathogenic bacteria associated with peri-implantitis require more than one antibiotic.15
Provided that mechanical and antiseptic protocols are followed prior to administering antibiotic therapy, it appears that shallow peri-implant infection may be successfully controlled using conventional antibiotics. Still open to question is whether deeper peri-implant lesions can be adequately treated nonsurgically using a combination of a local antibiotics and mechanical debridement. The decision to use adjunctive systemic antimicrobials should be made on an individual basis. Adequate debridement, good oral hygiene and maintenance care are still the most important strategies for successful treatment outcomes.
NANOPARTICLES AS ANTIBACTERIALS
Some metal-based antibacterials — silver Ag(I) and zinc, for example — are attractive alternatives to conventional antibiotics. Metal ions have chemical properties that inhibit bacterial growth. The unique binding, coordination chemistries and redox properties of metal-based antibacterials make development of bacterial resistance less likely, and suggest effectiveness across a broad bacterial spectrum. For example, Ag(I) and mercury have a long history of use as antibacterials, although fears of systemic toxicity have limited their use in recent years. Other metal ions, such as gold Au(III), palladium and platinum, have binding, coordination chemistries and redox properties that suggest they, too, would be effective antibacterial agents. Unfortunately, development of new metal-based antibacterials has been impeded due to previous controversies. Yet, given the increasing resistance of bacteria to antibiotics, metal-based antibacterials are a promising alternative. The minute size of nanoparticles gives them a larger surface area-to-volume ratio and they show antimicrobial effect at very low levels. In addition, metal-based nanoparticles can easily penetrate the bacterial cell membrane, resulting in rapid bactericidal activity. If systemic toxicity could be limited and therapeutic indices were optimized, metal ions and their associated compounds could emerge as a powerful new class of antibacterial agents.
Aside from difficulties in manufacturing (wherein nanoparticles tend to agglomerate), this class of metal nanoparticles has other inherent shortcomings. Titanium (Ti) particles can accumulate in the soft tissue via micro-motion and corrosion. Both Ag(I) ions and Ag(I) nanoparticles contribute to toxicity. These effects are due to reactive oxygen species (ROS) production. Such production can lead to disruption of various cellular processes, genotoxicity, lysosomal AcP activation, actin disruption, hemocyte phagocytosis stimulation and inhibition of Na-K-ATPase.16
One of the shortcomings of metal titanate materials can be turned around to its advantage. Metal titanates are known to discolor due to the ease of redox reactions. Their applications in direct restorative materials is thus limited. However, the compatibility of metal titanate with Ti implants make them a useful coating to fight peri-implantitis.
BACTERIAL RESISTANCE
The search for new, effective bactericidal materials is vital to combat drug resistance, and the application of nanoparticles is a promising solution to this problem. Given the bactericidal mode of nanoparticles, which is based on their specific physicochemical properties, most types can overcome at least one of the common mechanisms of bacterial resistance. In contrast to traditional antibiotics, nanoparticles’ minute size results in novel properties, such as greater interaction with cells due to a larger surface area-to-mass ratio, and versatile and controllable application.
In addition to the disruption of bacterial membranes — and given that biofilm plays a pivotal role in the development of bacterial resistance — hindrance of biofilm formation is an important action of nanoparticles. The structure of biofilm offers protection to the embedded microorganisms, shielding them from most antibiotics and providing a breeding ground for frequent resistance mutations and the exchange of these mutations among various bacterial cells. Studies have shown that many nanoparticles can prevent or overcome biofilm formation. Additionally, the specific particulate shape of nanoparticles effects biofilm destruction; for example, nanoparticles with a rod-like shape are more effective than those with a spherical shape.
NANOPARTICLE METAL TITANATE COMPLEXES
The growing resistance of microbes to current antimicrobial agents creates a unique niche for using metal titanate complexes to fight pathogenic microbes; this is due to the ability of such complexes to deliver metal ions in a controlled manner. Nanoparticle metal titanate has been successfully developed as a novel antibacterial agent.17–21
Titanates are insoluble particulate compounds of Ti and oxygen with crystalline surfaces that bind metal ions.17 Titanates combined with metal ions are effective as broad-spectrum antibiotics, antifungals and anti-proliferative agents due to their redox potential.20 These compounds can also serve to remove metal ions from biologic environments, as well as successfully deliver them in a localized fashion — without causing systemic toxicity. Additionally, metal titanates have a greater affinity for more highly positively charged species (M3+) than for less positively charged species (M2+). Therefore, competition between protons, sodium ions and metal ions with titanate binding sites makes the successful delivery of metals to tissues probable in biological settings.21
It has been shown that nanoparticle metal titanate complexes are effective at inhibiting oral bacterial growth, resulting in more effective ion-exchange characteristics.12 The antibacterial mechanisms of nanoparticles are still poorly understood, however. In contrast, the antimicrobial mechanism of traditional antibiotics is usually relatively simple, which is partly responsible for the emergence of bacterial resistance. Unlike traditional antibiotics, nanoparticles combat microbes via multiple mechanisms that are active simultaneously. Thus, a microbe is unlikely to adapt to several lines of attack at once, which makes it more difficult to develop resistance to nanoparticles than traditional antibiotics.
The use of metal ions as medicaments is made possible by their redox potential, geometries, thermodynamic and kinetic properties, and other intrinsic properties that make them effective as therapeutic agents.22,23 Metal ions have been used in medicinal contexts in the treatment of cancers, inflammation and arthritis, among other pathologies.22 The increasing use of nanoparticles in medicine has led to a growing number of studies exploring their potential antibacterial mechanisms. For example, metal nanoparticles can change the metabolic activity of bacteria. This represents a huge advantage in terms of eliminating bacteria to cure diseases. The ability of nanoparticles to enter oral biofilm also provides a practical method to inhibit biofilm formation based on the Ag(I)-inhibited expression of genes.
Nanoparticles need to be in contact with bacterial cells to achieve their antibacterial function. Accepted forms of contact include electrostatic attraction, van der Waals forces, and receptor-ligand and hydrophobic interactions. After crossing bacterial membranes, nanoparticles affect metabolic pathways, influencing the shape and function of the cell membrane. Thereafter, they interact with the basic components of bacterial cells, such as deoxyribonucleic acid (DNA), lysosomes, ribosomes and enzymes, leading to oxidative stress, heterogeneous alterations, changes in cell membrane permeability, electrolyte balance disorders, enzyme inhibition, protein deactivation and changes in gene expression.
Recently, a genetic approach was undertaken in an attempt to understand the mechanism of bacterial growth inhibition by metal ion species.24 This is important to verify that metal ion species will not cause significant adverse reactions within host cells. Nanoparticle-mediated inhibition of bacterial protein and DNA synthesis, as well as nanoparticle-mediated regulation of the expression of metabolic genes, were investigated. Results of this study indicated that Lactobacillus casei did not exhibit resistance to the Au(III)-MST complex, whereas Streptococcus mutans was able to grow in the presence of up to 200 mg/L Au(III)-MST. The mechanism underlying the antimicrobial effect of Au(III)-MST on L. casei may be via the regulation of srtA1 and fbpA genes, and on S. mutans via the regulation of the Pac gene. These findings represent a small step toward understanding the ultimate mechanisms of nanoparticle inhibition of bacteria.
EXPERIMENTAL APPROACHES
One of the previously suggested causal factors of peri-implantitis is Ti corrosion. Specifically, electrical conductivity of Ti implants plays a crucial role in the extent of Ti corrosion. In volunteer patients, quantities of leached Ti were measured, indicating a combined effect of electrochemical corrosion and mechanical wear. Presumably, this results from electrons reducing anaerobic zone flow through the implant into the oxygenated zone.25 Interrupting this electrical current significantly reduced Ti corrosion.26 Based on these findings, an implant concept was proposed consisting of interrupted Ti antimicrobial metal bands deposited onto ceramic implants (Figure 2). Such ceramic implants can be modified by three-dimensional printing and, thus, this hybrid/composite implant combines the benefits of two materials. Although nonconductive and noncorrosive zirconia implants exist, they lead to increased bone loss when compared to Ti implants.
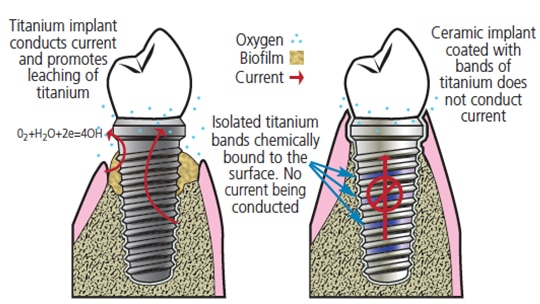
disease involves the use of ceramic implants coated with interrupted bands of
antimicrobial metal titanate (UW provisional patent granted in 2016).27
With a zirconia-Ti composite implant, bone readily integrates with the Ti surface, increasing the longevity and stability of the restoration. Ceramic is electrically nonconductive, chemically resistant and mechanically strong. Other salient characteristics of this composition include:
- Ceramics are not widely used in the United States, but are applied more broadly in Europe.
- The proposed implant is fabricated from solid zirconia coated with electrically insulated Ti bands chemically bound to the surface. Bone can anchor to these titanium areas, but electrons cannot flow between them.
- This is an improvement over other designs that are not “true” composites, for which the joining of Ti to ceramic becomes a breaking point when under pressure. In comparison, Au(III) and Ag(I) nanoparticles have been used on surfaces modified to create a combination of Ag(I), Ti dioxide and hydroxyapatite (HA) nanocoatings. Thus, one solid implant addresses the issue noted above.
CONCLUSION
One of the most common causes of implant failure is peri-implantitis, which is caused by the same bacterial biofilm that causes caries and periodontal disease. Researchers are seeking to disrupt bacterial adherence to implants by modifying implant surface topography. Toward this goal, Au(III) and Ag(I) nanoparticles have been used on surfaces modified to create a combination of Ag(I), Ti dioxide and hydroxyapatite (HA) nanocoatings. Coated implants were found to have antibacterial properties and HA biocompatibility — with no apparent compromise to structural integrity. A new method of coating ceramic implants with stripes was also introduced.27 It must be cautioned that no significant studies have been performed at this time to demonstrate either in vitro or in vivo efficacy, particularly against the pathogenic bacteria associated with peri-implantitis. That noted, metal titanate nanoparticles may represent a new tool for managing peri-implant disease.
Acknowledgment: The authors wish to thank Dr. Hollie Walsh for her editorial advice, and the National Institute of Dental and Craniofacial Research Grant No. RO1DE021373-01, and WDS endowment for funding various aspects of this work.
Disclosure: Relevant to this manuscript, Daniel C. Chan holds U.S. patent No. US-2012-0156145-A1 “Use of Titanium-Based Materials as Bactericides.” Chan and Alexander E. Pozhitkov are holders of the UW provisional patent No. 47613 “Striped-spangle Dental Implant.”
REFERENCES
- Sullivan RM. Implant dentistry and the concept of osseointegration: a historical perspective. J Calif Dent Assoc. 2001;29:737–745.
- American Academy of Implant Dentistry. What Are Dental Implants? Available at: aaid-implant.org/dental-implants/what-are-dental-implants/. Accessed September 18, 2018.
- Berglundh T, Persson L, Klinge B. A systematic review of the incidence of biological and technical complications in implant dentistry reported in prospective longitudinal studies of at least 5 years. J Clin Periodontol. 2002;29(Suppl 3):197–212; discussion 232–293.
- Atieh MA, Alsabeeha NH, Faggion CM Jr, Duncan WJ. The frequency of peri-implant diseases: a systematic review and meta-analysis. J Periodontol. 2013;84:1586–1598.
- Caton JG, Armitage G, Berglundh T, et al. A new classification scheme for periodontal and peri-implant diseases and conditions — introduction and key changes from the 1999 classification. J Clin Periodontol. 2018;45:45(Suppl 20):S1–S8.
- Schwarz F, Derks J, Monje A, Wang HL. Peri-implantitis. J Clin Periodontol. 2018;45(Suppl 20):S246–S266.
- Buser D, Janner SF, Wittneben JG, Brägger U, Ramseier CA, Salvi GE. 10-year survival and success rates of 511 titanium implants with a sandblasted and acid-etched surface: a retrospective study in 303 partially edentulous patients. Clin Implant Dent Relat Res. 2012;14:839–851.
- Konstantinidis IK, Kotsakis GA, Gerdes S, Walter MH. Cross-sectional study on the prevalence and risk indicators of peri-implant diseases. Eur J Oral Implantol. 2015;8:75–88.
- Daubert DM, Weinstein BF, Bordin S, Leroux BG, Flemmig TF. Prevalence and predictive factors for peri-implant disease and implant failure: a cross-sectional analysis. J Periodontol. 2015;86:337–347.
- Daubert DM. Titanium corrosion is a modifier of peri-implant health. Available at: digital.lib.washington.edu/researchworks/bitstream/handle/1773/38565/Daubert_washington_0250E_16831.pdf?sequence=1&isAllowed=y. Accessed September 18, 2018.
- Norowski PA Jr, Bumgardner JD. Biomaterial and antibiotic straegies for peri-implantitis: a review. J Biomed Mater Res B Appl Biomater. 2009;88:530–543.
- Chan HL, Lin GH, Suarez F, MacEachern M, Wang HL. Surgical management of peri-implantitis: a systematic review and meta-analysis of treatment outcomes. J Periodontol. 2014;85:231–238.
- Froum SJ, Froum SH, Rosen PS. A regenerative approach to the successful treatment of peri-implantitis: a consecutive series of 170 implants in 100 patients with 2- to 10-year follow-up. Int J Periodontics Restorative Dent. 2015;35:857–863.
- Javed F, Alghamdi AS, Ahmed A, Mikami T, Ahmed HB, Tenenbaum HC. Clinical efficacy of antibiotics in the treatment of peri-implantitis. Int Dent J. 2013;63:169–176.
- Rams TE, Feik D, Mortensen JE, Degener JE, van Winkelhoff AJ. Antibiotic susceptibility of periodontal Streptococcus constellatus and Streptococcus intermedius clinical Isolates. J Periodontol. 2014;85:1792–1798.
- Priyadarsini S, Mukherjee S, Mishra M. Nanoparticles used in dentistry: a review. J Oral Biol Craniofac Res. 2018;8:58–67.
- Eiampongpaiboon T, Chung WO, Bryers JD, Chung KH, Chan D. Antimicrobial activity of gold-titanates on Gram-positive cariogenic bacteria. Acta Biomater Odontol Scand. 2015;1:51–58.
- Eiampongpaiboon T, Chung WO, Chan DC, Bryers JD, Chung KH. Effects of gold-titanate on Streptococcus mutans and Lactobacillus casei viability. Conference paper. AADR Annual Meeting and Exhibition 2014.
- Chan DC, Eiampongpaiboon T, Deng S. Nanotechnology in dentistry — A review of filler development and antibacterial applications. J Cosmetic Dent. 2015;31:86–94.
- Use of Titanium-Based Materials as Bactericides. Available at: patents.google.com/patent/US20120156145. Accessed September 18, 2018.
- Chen YW, Drury JL, Chung WO, Hobbs DT, Wataha JC. Titanates and titanate-metal compounds in biological contexts. Int J Med Nano Res. 2015;2:009.
- Su YH, Yin ZF, Xin HL, et al. Optimized antimicrobial and antiproliferative activities of titanate nanofibers containing silver. Int J Nanomedicine. 2011;6:1579–1586.
- Bruijnincx PC, Sadler PJ. New trends for metal complexes with anticancer activity. Curr Opin Chem Biol. 2008;12:197–206.
- Theda L, Kim P, Chan D, Chung WO. Titanate-metal complex as a novel antimicrobial in dentistry. Dent Oral Biol Craniofac Res. 2018;1:5–6.
- Safioti LM, Kotsakis GA, Pozhitkov, AE, Chung WO, Daubert DM. Increased levels of dissolved titanium are associated with peri-implantitis — a cross-sectional study. J Periodontol. 2017;88:436–442.
- Pozhitkov AE, Daubert D, Brochwicz Donimirski A, et al. Interruption of electrical conductivity of titanium dental implants suggests a path towards elimination of corrosion. PLoS ONE. 2015;10:e0140393.
- UW provisional patent No. 47613 Striped-spangle Dental Implant.
Featured image by AYDINOZON/ISTOCK/GETTY IMAGES PLUS
From Decisions in Dentistry. October 2018;4(10):35–38.