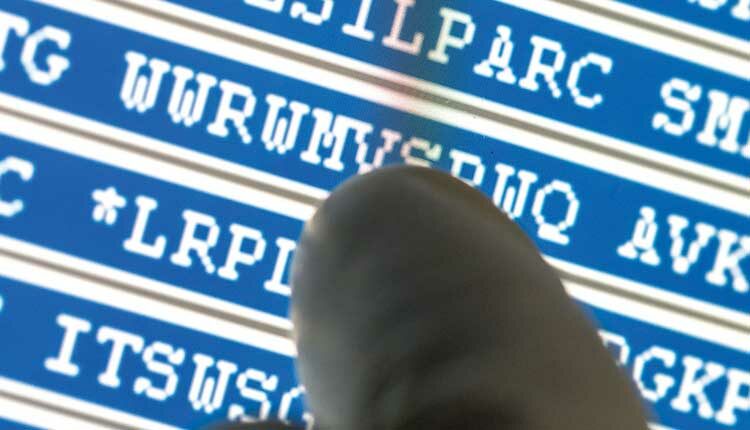
Therapeutic Uses for Antimicrobial Peptides in Oral Healthcare
This class of therapeutics may provide effective treatment for oral infections, such as caries, periodontal disease, oral mucosal diseases, and oral cancer.
This course was published in the December 2021 issue and expires December 2024. The authors have no commercial conflicts of interest to disclose. This 2 credit hour self-study activity is electronically mediated.
EDUCATIONAL OBJECTIVES
After reading this course, the participant should be able to:
- Discuss the role of microbial pathogens in the caries process.
- Define antimicrobials peptides (AMPs).
- Explain applications for synthetic AMPs in treating caries.
Dental caries is frequently researched by oral health professionals in hopes of finding new treatments, as it remains one of the most prevalent infectious diseases and global public health issues.1 Caries is caused by an accumulation of plaque-associated microbes that produce demineralizing acidic by-products that can damage tooth structure, including enamel, dentin and cementum. The development of caries lesions can be attributed to continued ecological imbalance of acid-producing pathogens and exposure to their derivatives over an extended time.2 Research in this area is leading to new therapies to prevent and manage caries, including clinical applications for antimicrobial peptides (AMPs), which are the subject of this report.
The oral cavity contains one of the most diverse and populated microbiota in the human body, with more than 700 bacterial species identified, not including fungi, viruses and protozoa.3 The most well-studied cariogenic bacteria residing in oral biofilm are Streptococcus mutans, Streptococcus sobrinus, Actinomyces and Lactobacilli. Other pathogenic species that can contribute to caries include fungi, specifically Candida albicans.4 S. mutans is the primary bacterial species responsible for the development of caries lesions.5 In addition, low pH, reduced salivary flow, and/or routine consumption of carbohydrates can exacerbate lesion progression. Without protective factors, such as salivary buffers and fluoride exposure, bacterial metabolism can remain unchecked.2
Because the complete elimination of plaque biofilm is difficult to achieve, alternative approaches to reducing pathogenic flora have been developed.6 Anticaries therapies — including various antimicrobial agents, such as fluoride, triclosan, chlorhexidine and phenols — are used to reduce bacterial load and prevent biofilm formation.7,8 Various delivery vehicles, such as trays and varnishes, are utilized for in-office treatment. More recently, silver diamine fluoride has been accepted as an off-label treatment in arresting caries lesions.9 Fluoride, triclosan and phenols are commonly used in over-the-counter oral hygiene products to provide preventive effects against caries during self-care.10
Although current methodologies can be effective in controlling biofilm accumulation and preventing demineralization, they are not without drawbacks. Some individuals are opposed to the use of fluoride and triclosan due to safety concerns. In addition, prolonged chlorhexidine use raises the risk of hypersensitivity and discoloration of hard and soft oral tissue.11 While phenolics provide antimicrobial and antigingivitis properties, they may cause irritation and burning sensations.12 These therapies may also disrupt the microbial balance of the oral cavity when used for extended periods.13 Additionally, due to increased drug resistance, conventional systemic antibiotics are not commonly prescribed to treat oral diseases.14,15 Further, oral biofilms are already resistant to antibiotics such as amoxicillin and metronidazole.6 As such, research has focused on developing alternative therapeutics — such as AMPs — which may offer an effective treatment for oral infections, including caries, periodontal disease, oral mucosal diseases, and oral cancer.16
NATURAL DEFENSE MECHANISM
Occurring naturally in multicellular organisms, AMPs consist of amino acid sequences of varying lengths and are found in the epithelial lining, blood and lymphatic tissues.17 They are, in fact one of the first defenses against foreign bodies. They also play an important role in the oral cavity. Produced in response to infections, AMPs bind to and neutralize invading microorganisms.18 Currently, 3273 AMPs are known to exist, and derive from sources such as bacteria, fungi, plants and animals, according to the Antimicrobial Peptide Database.19 This number will continue to grow as more are discovered or created. They can induce antibacterial, antibiofilm, antifungal, antiviral, anticandida, anti-inflammatory, antitumor, and/or wound healing effects.2,18
Due to their broad-spectrum antibacterial properties, AMPs can target specific Gram-positive and Gram-negative bacteria. They have specialized mechanisms to control bacteria, fungus and virus populations.20,21 Research has shown that resistance to various AMPs is rare, which makes them a promising therapeutic candidate for infectious diseases, including caries.2,16,22 Investigators are now working on developing synthetic AMPs. Designing artificial peptides allows researchers to isolate and enhance specific desired characteristics to provide additional benefits, such as increased stability.23,24 As AMPs can be readily applied to the oral cavity, numerous opportunities are available to develop prevention and treatment modalities for in-office and self-care use in caries management.25
NATURAL ANTIMICROBIAL PEPTIDES IN THE ORAL CAVITY
As noted, human oral AMPs are naturally occurring and provide the following benefits:26
- They defend against pathogens
- Their action repairs cellular tissues
- AMPs help maintain the oral cavity
Salivary proteins, such as histatins, defensins and cathelicidins, are well-known AMPs. These salivary proteins have vastly different structures in their amino acid sequences and sizes, not only between each other, but also within each type.2,16,27 Histatins, defensins and cathelicidins play a protective role in oral health.25 Numerous histatin peptide sequences are secreted from the submandibular and parotid salivary glands. Some histatin peptides have fungicidal properties that can kill C. albicans.26 Other histatins aid in enamel protection by binding to hydroxyapatite and preventing acid erosion.2 Cathelicidins and defensins have displayed broad-spectrum antimicrobial activity against oral pathogens, such as Gram-negative and Gram-positive bacteria, fungi and viruses.27,28 Cathelicidins elicit antimicrobial activity and antisepsis effects. They can trigger distinct defense systems and help repair wounds. Cathelicidins can also promote cytolysis.27 Research is underway on the use of defensins due to their ability to kill targeted microorganisms and their low risk of creating pathogenic resistance.29
As part of the innate immune response, production of natural AMPs increases during diseased states.16 While studies have shown several human AMPs to be bactericidal to S. mutans and others to be fungicidal to C. albicans, natural AMPs are not able to completely eradicate these pathogens.25,30 They primarily control overgrowth of harmful microorganisms and sustain the balance of oral microflora.30 Naturally occurring AMPs are limited due to their instability in the ever-changing oral environment.23,31 They are susceptible to proteases — enzymes that break down proteins. As a result, they generally have short half-lives due to their rapid deterioration. Natural AMPs, therefore, have low bioavailability.2,24 These limitations create challenges to the use of natural AMPs in managing oral infections.
SYNTHETIC ANTIMICROBIAL PEPTIDES
Synthetic AMPs may offer an alternative to traditional antimicrobial and antibiotic treatments. Researchers have identified several selectively targeted AMPs (STAMPs) that can be utilized against S. mutans. Specific STAMPs have the potential to directly kill S. mutans within the diverse plaque biofilm.32 In one study, 43 synthetic AMPs with different mechanisms successfully inhibited the growth of S. mutans.25 Some synthetics also demonstrated the ability to increase attraction for hydroxyapatite and promote remineralizing effects on dentition.25 Certain AMPs modeled from histatins have high biodegradability and demonstrate efficacy against C. albicans.26,33 In addition, plaque formation may be impeded by some synthetic AMPs due to their competitive blockage of bacterial adhesion.34
Researchers are focused on developing synthetic AMPs with improved stability and beneficial modifications of natural AMP structures.16 When designing synthetic peptides, researchers are aiming to produce safe, effective and stable AMPs that can be targeted for specific uses in oral healthcare.23,24 They can be created using three methods:
- Mimesis
- New design synthesis
- Fusion of peptides
Mimesis mimics natural AMPs by using their sequence templates to create improved artificial peptides.25 New design synthesis uses innovative formulations to produce original antimicrobial agents.35 Fusion of peptides takes functional sequences of existing peptides and increases the specificity and/or activity of synthesized AMPs.2 They can also be improved by including additional amino acids or removing sequences to strengthen the peptide’s action.35 An important determination in the success of synthesized AMPs is their ability to work together to enhance each other’s effects.18
In order for synthetic AMPs to become more common in therapeutic applications, stability must be improved so they can better withstand degradation in the oral environment.35 Once greater stability and effectiveness are achieved, other challenges may include the cost of production, obtaining authorization from governing bodies, and potential consumer resistance of a product labeled “synthetic.”2
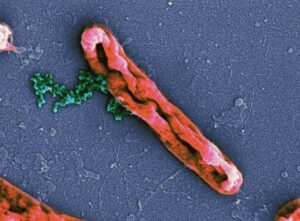
MECHANISMS OF ACTION
Diverse in their mechanism of action, AMPs can kill cariogenic bacteria, suppress the formation of plaque biofilm, and inhibit enzymes and protein synthesis, among other activities.5,16,36,37 Some, such as cathelicidins, can differentiate healthy host cell membranes and not act upon them.27
Many AMPs adhere to cell membranes, thereby damaging and puncturing specific cells.27 In addition, they can penetrate cell walls and cytoplasmic membranes of bacteria to form channels, which lead to leakage of the cells’ contents and disintegration of the membrane (Figure 1).16,27 The mechanisms of AMPs’ attachment and insertion into cell membranes are categorized by the following models:38
- Barrel-stave
- Toroidal-pore
- Carpet
- Detergent-like
Barrel-stave and toroidal-pore mechanisms both create gaps, causing membranes to become more permeable, thinner and/or leak.27,38 Carpet and detergent-like mechanisms affect membranes by disrupting them and reducing cell resistance and density.38
Alternatively, adherence to cell walls can also cause modification of bacterial structures and curvature.39 After adhering to a lipid bilayer, AMPs can insert themselves into the outside layer of pathogens and destabilize cell membranes.34 In Gram-negative bacteria, they can move through the outer membrane and the inner cell wall into the cytoplasm.27 Once AMPs have entered a cell, if not cytolytic, they can further selectively disrupt protein synthesis and prevent cell wall formation. Some AMPs bind to intracellular organelles and inhibit enzyme activity.40
A major therapeutic advantage of AMPs is their specificity. They can kill pathogens without damaging surrounding host environments.16 Studies have reported both natural and synthetic AMPs are effective against S. mutans due to their inhibitory and bactericidal qualities.25 Furthermore, AMPs have synergistic abilities whereby acting together enables lower concentrations to be more effective.18
CONCLUSION
A promising approach to oral disease management, AMPs are being studied as alternative methods for prevention and treatment of caries, periodontal disease and oral cancer. In caries management, AMPs have the ability to specifically target and eliminate caries-causing bacteria. Naturally occurring AMPs are already found in saliva and function as part of the protective factors against disease-causing pathogens. While natural AMPs usually have short half-lives and rapidly deteriorate in the oral cavity, the development of synthetic AMPS may overcome these limitations.
While numerous laboratory studies demonstrate the effectiveness of AMPs against S. mutans and other pathogenic microbes, including C. albicans, further research is needed. Ultimately, whether natural or synthetic, AMPs hold considerable potential for use in managing caries and other oral infections.
References
- Žemaitienė M, Grigalauskienė R, Vasiliauskienė I, Saldūnaitė K, Razmienė J, Slabšinskienė E. Prevalence and severity of dental caries among 18-year-old Lithuanian adolescents. Medicina. 2016;52:54–60.
- Pepperney A, Chikindas ML. Antibacterial peptides: opportunities for the prevention and treatment of dental caries. Probiotics Antimicrob Proteins. 2011;3:68.
- Deo PN, Deshmukh R. Oral microbiome: unveiling the fundamentals. J Oral Maxillofac Pathol. 2019;23:122–128.
- Struzycka I. The oral microbiome in dental caries. Pol J Microbiol. 2014;63:127–135.
- Ding Y, Wang W, Fan M, et al. Antimicrobial and anti-biofilm effect of Bac8c on major bacteria associated with dental caries and Streptococcus mutans biofilms. Peptides. 2014;52:61–67.
- Marsh PD. Dental plaque as a microbial biofilm. Car Res. 2004;38:204–211.
- Kalesinskas P, Kačergius T, Ambrozaitis A, Pečiulienė V, Ericson D. Reducing dental plaque formation and caries development. A review of current methods and implications for novel pharmaceuticals. Stomatologija. 2014;16:44–52.
- Marsh PD. Controlling the oral biofilm with antimicrobials. J Dent. 2010;38:S11–S15.
- Horst JA, Ellenikiotis H, Milgrom PL. UCSF protocol for caries arrest using silver diamine fluoride: rationale, indications and consent. J Calif Dent Assoc. 2016;44:16–28.
- Ten Cate JM. Novel anticaries and remineralizing agents. J Dent Res. 2012;91:813–815.
- Pemberton MN, Gibson J. Chlorhexidine and hypersensitivity reactions in dentistry. Br Dent J. 2012;213:547–550.
- Wilkins EM, Wyche CJ, Boyd LD, Mallonee LF. Clinical Practice of the Dental Hygienist. 13th ed. Burlington, Mass: Jones & Bartlett Learning; 2021:1042–1043.
- Marsh PD, Head DA, Devine DA. Ecological approaches to oral biofilms: control without killing. Caries Res. 2015;49(Suppl 1):46–54.
- Angebault C, Andremont A. Antimicrobial agent exposure and the emergence and spread of resistant microorganisms: issues associated with study design. Eur J Clin Microbiol Infect Dis. 2012;32:581–595.
- Chon SY, Doan HQ, Mays RM, Singh SM, Gordon RA, Tyring SK. Antibiotic overuse and resistance in dermatology. Dermatol Ther. 2012;25:55–69.
- Niu JY, Yin IX, Mei ML, Wu WK, Li QL, Chu CH. The multifaceted roles of antimicrobial peptides in oral diseases. Mol Oral Microbiol. 2021;36:159–171.
- Di Somma A, Moretta A, Canè C, Cirillo A, Duilio A. Antimicrobial and antibiofilm peptides. Biomolecules. 2020;10:652.
- Piotrowska U, Sobczak M, Oledzka E. Current state of a dual behaviour of antimicrobial peptides — therapeutic agents and promising delivery vectors. Chem Biol Drug Des. 2017;90:1079–1093.
- University of Nebraska Medical Center. Antimicrobial Peptide Database. Available at: https://aps.unmc.edu/AP. Accessed November 2, 2021.
- Aoki W, Kuroda K, Ueda M. Next generation of antimicrobial peptides as molecular targeted medicines. J Biosci Bioeng. 2012;114:365–370.
- Zhang J, Chen C, Chen J, et al. Dual mode of anti-biofilm action of G3 against Streptococcus mutans. ACS Appl Mater Interfaces. 2020;12:27866–27875.
- da Silva BR, de Freitas VA, Nascimento-Neto LG, et al. Antimicrobial peptide control of pathogenic microorganisms of the oral cavity: A review of the literature. Peptides. 2012;36:315–321.
- Bechinger B, Gorr SU. Antimicrobial peptides: mechanisms of action and resistance. J Dent Res. 2016;96:254–260.
- Shao C, Zhu Y, Lai Z, Tan P, Shan A. Antimicrobial peptides with protease stability: progress and perspective. Future Medicinal Chemistry. 2019;11:2047–2050.
- Niu JY, Yin IX, Wu WK, Li QL, Mei ML, Chu CH. Antimicrobial peptides for the prevention and treatment of dental caries: a concise review. Arch Oral Biol. 2021;122:105022.
- Khurshid Z, Najeeb S, Mali M, et al. Histatin peptides: pharmacological functions and their applications in dentistry. Saudi Pharm J. 2017;25:25–31.
- Kościuczuk EM, Lisowski P, Jarczak J, et al. Cathelicidins: family of antimicrobial peptides. A review. Mol Biol Rep. 2012;39:10957–10970.
- Khurshid Z, Zafar MS, Naseem M, Khan RS, Najeeb S. Human oral defensins antimicrobial peptides: a future promising antimicrobial drug. Curr Pharm Des. 2018;24:1130–1137.
- Gorr SU, Abdolhosseini M. Antimicrobial peptides and periodontal disease. J Clin Periodontol. 2011;38:126–141.
- Van Nieuw Amerongen A, Bolscher JG, Veerman EC. Salivary proteins: protective and diagnostic value in cariology? Caries Res. 2004;38:247–253.
- Lei J, Sun L, Huang S, et al. The antimicrobial peptides and their potential clinical applications. Am J Transl Res. 2019;11:3919–3931.
- Guo L, Edlund A. Targeted antimicrobial peptides: a novel technology to eradicate harmful Streptococcus mutans. J Calif Dent Assoc. 2017;45:557–564.
- do Nascimento Dias J, de Souza Silva C, de Araújo AR, et al. Mechanisms of action of antimicrobial peptides ToAP2 and NDBP-5.7 against Candida albicans planktonic and biofilm cells. Sci Rep. 2020;10:10327.
- Ito T, Ichinosawa T, Shimizu T. Streptococcal adhesin SspA/B analogue peptide inhibits adherence and impacts biofilm formation of Streptococcus mutans. PLoS One. 2017;12:e0175483.
- Zhang LY, Fang ZH, Li QL, Cao CY. A tooth-binding antimicrobial peptide to prevent the formation of dental biofilm. J Mater Sci Mater Med. 2019;30:45.
- Nguyen LT, Haney EF, Vogel HJ. The expanding scope of antimicrobial peptide structures and their modes of action. Trends Biotechnol. 2011;29:464–472.
- Wang Z, de la Fuente-Núñez C, Shen Y, Haapasalo M, Hancock RE. Treatment of oral multispecies biofilms by an anti-biofilm peptide. PLoS One. 2015;10:e0132512.
- Chang WK, Wimley WC, Searson PC, Hristova K, Merzlyakov M. Characterization of antimicrobial peptide activity by electrochemical impedance spectroscopy. Biochim Biophys Acta. 2008;1778:2430–2436.
- Bertelsen K, Dorosz J, Hansen SK, Nielsen NC, Vosegaard T. Mechanisms of peptide-induced pore formation in lipid bilayers investigated by oriented 31P solid-state NMR spectroscopy. PLoS One. 2012;7:e47745.
- Nicolas P. Multifunctional host defense peptides: intracellular-targeting antimicrobial peptides. FEBS J. 2009;276:6483–6496.
From Decisions in Dentistry. December 2021;7(11):26-28, 31.