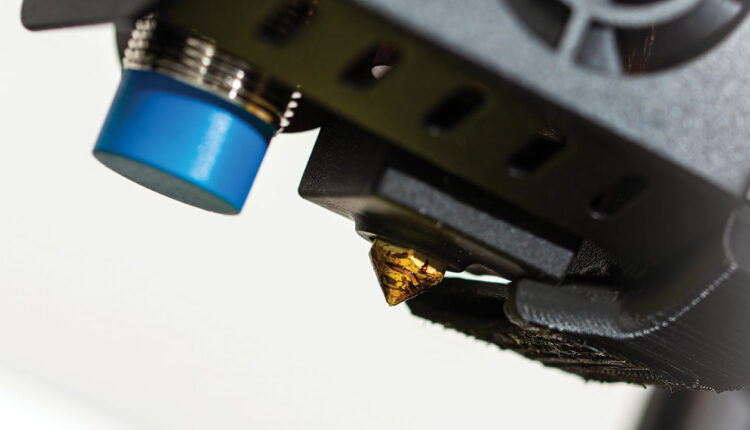
How 3D Printing Is Shaping the Future of Dental Care
The advancement of three-dimensional printing in dentistry offers a more efficient, cost-effective, and innovative approach to dental treatments, paving the way for complex, customized solutions that traditional methods can’t achieve.
Advancement of digital technology in dentistry has allowed for more efficient and innovative treatment modalities compared to conventional solutions.1 Computer-aided design and computer-aided manufacturing (CAD/CAM) materials, such as the new generations of high-strength ceramics, metal alloys, and particle-filled hybrid composites, have allowed a large variety of restorative options and clinical indications in fixed prosthodontics.2 Some technologies (eg, milling), however, require expensive equipment, waste a significant amount of material, and are unable to manufacture complex objects due to the size of the tooling (eg, size of cutting burs or millable disk).3,4
Three-dimensional (3D) printing, or additive manufacturing, involves curing successive layers of printed materials such as plastics, resins, and metals. Additive manufacturing emerged in the mid 1980s.5 Since then, 3D printing has made its way into the dental field. Initially, it was primarily used in the production of models for diagnostic and planning purposes. As the technology advanced, 3D printing capabilities expanded, allowing for the manufacturing of more precise and accurate guides, models, impression trays, and prostheses of all kinds, with improved efficiency.6 Today, 3D printing is highly desirable due to its ability to reduce waste and produce highly complex objects in a cost-effective manner.7
Types of Printers and Materials
Several additive manufacturing technologies are available in dentistry, including stereolithography (SLA), digital light processing (DLP), selective laser melting (SLM), and material jetting (MJ).
SLA uses a laser beam to cure light-sensitive resin materials by scanning layer by layer. Due to the small surface area of the beam, this technology is known for its high precision and surface finish.
DLP technology also cures light-sensitive resin materials. However, it works by shining a pattern on a screen corresponding to the pattern of each layer at once to cure the resin layer by layer. Both SLA and DLP technologies typically use light in the blue or ultraviolet spectrum, and they are great solutions for printing fixed and removable prostheses, as well as creating dental models, dental splints, and surgical guides.
In SLA and DLP, the build platform is lowered on the vertical Z-axis and is immersed in the bottom of a vat filled with liquid resin monomer. A space is established between the platform and the bottom of the vat to allow for the monomer to flow in between and then the photopolymerized monomer is light cured. After each printed layer, the build platform moves up to make room for the next layer, and the cycle repeats.8
The monomers used for DLP and SLA printers should have low to moderate viscosity. Acrylate monomer resins are most widely used in dentistry with these technologies. Diluents, such as triethylene glycol diacrylate, are added to reduce the viscosity combined with photoinitiators to allow for light polymerization.
Inhibitors are also added to prevent cross linking during storage. If fillers are added to increase the mechanical properties of a given resin, viscosity can be negatively affected. To ensure a homogenous dispersion of fillers during the printing process, methods, such as heating, shaking, stirring, and tilting of the resin, may be used.7
MJ uses inkjet technology to deposit photopolymerized resin, which is then cured with light. Due to the nature of depositing material rather than curing layers inside a vat of resin, this technology is known for its ability to print objects made of different materials and colors, making it ideal for representative models and prototypes.9
SLM also uses a laser beam to melt and fuse polymer powder into solid objects after cooling. The material spectrum ranges from plastics to ceramics to metals. In dentistry, this technology is typically used for metal manufacturing, to produce surgical guides, implants, crown and bridge copings, and removable partial denture frameworks.9
Advantages and Disadvantages
Considering the advantages of 3D printing, its increased popularity is no surprise. This is because it can produce less waste as it uses only the material required to produce the desired object, rather than having to mill it from a bigger block. It is also capable of producing complex objects as it is not limited by the size of any tooling. Lastly, the cost involved in 3D printing in the short- and long-term is more economically effective compared to other digital methods like milling.7
However, 3D printing does come with some drawbacks. The learning curve involved in designing and manufacturing may deter some practitioners. This method can be a slow process, as more complex objects may need more intricate support systems to ensure printability, require more layers to be printed, and result in a considerable amount of time being lost between layers, as each layer has to be peeled from the bottom of the resin vat prior to the manufacturing of the following layer. Moreover, the range of printable materials is still limited compared to other manufacturing methods such as milling. Esthetic appearance, wear resistance, and dimensional accuracy of 3D-printing technologies and materials also pose some issues; more research is needed in these areas to expand the progression of this field.10
Recent Developments
One major development in the field of 3D printing is continuous liquid production technology (CLIP). This technology is designed to reduce printing speeds by exploiting the concept of oxygen-inhibition of photopolymerized resin. At the bottom of the resin vat, CLIP uses an oxygen-permeable window, introducing a layer of oxygen at the bottom of the vat. Oxygen inhibition of the photopolymerized resin at the window interface means that the printed layer will not stick to the bottom of the resin vat, removing the need for slow peeling motions between each layer. The result is a continuous printing of the object as the build platform raises after the light projection of each layer.7,11
Another area of development is minimizing the gap of material compatibility. The printing of ceramic materials has always been a desired outcome of additive manufacturing development. For example, zirconia is a widely used material for crowns and fixed dental prostheses. Lithography-based ceramic manufacturing (LCM) is a common technology used to print metal-oxides, and lithium disilicate ceramics. It works by photopolymerizing a ceramic powder suspension in photopolymerizable resin. In this method, the resin acts like a binder, which has to be removed by pyrolysis (debinding), allowing for ceramic components to fuse together to reach the final properties and shape. More research is needed to determine the accuracy and physical properties of this method, but current research is promising.12–14
Another area of development is 4D printing, which adds time as the fourth dimension. Materials printed with this technology allow for dimensional changes, in that the product moves over time based on certain stimuli such as electric, magnetic, radiation, or moisture. For example, 4D printing may allow for the fabrication of orthodontic systems that provide the desired results over time. Alternatively, it can be used in dental and surgical devices, such that these devices may adjust for age, procedure, and daily activities depending on specific patient needs. All of these developments need more research before clinical use is made practically and economically possible.15,16
Case Report
A 65-year-old woman presented with extensive dental wear in the form of attrition and erosion, with a shortened maxillary arch. Medically, the patient was diagnosed with scleroderma associated with xerostomia, leading to high caries risk, with progressive and developing limited range of motion secondary to her scleroderma, making oral hygiene challenging, especially posteriorly.
Following the discussion about alternatives to treatment, and the relative risks and benefits of each option with the patient, the maxilla was planned for implant-supported and retained full-arch splinted reconstruction. Due to the patient’s xerostomia and her desire for fixed prostheses in all stages of the treatment, immediate implant placement and loading were the goals. As a result, the need for intricately designed guides and prostheses was essential. With its ability to allow for complex objects, 3D printing was key to the success of this approach.
For this patient, bone reduction was necessary to provide a tissue-prosthetic junction above her high smile line secondary to a hypermobile lip (Figure 1). Therefore, bone reduction and implant placement guides were designed to support surgical and prosthetic treatment. The bone-borne bone reduction guide was designed to allow for alveoloplasty, followed by a magnetically stackable implant placement guide. A magnetically stackable immediate-load prosthesis uses the planned implant locations for immediate loading (Figure 2).
Manufacturing such complex geometries of magnet beds, tubes, and intricate patrices and matrices would be challenging via milling due to limitations related to bur size and limited axes of rotation. Figures 3 through 5 demonstrate the magnetically stackable steps in sequence, with the implants being placed, followed by the planned angle-correcting screw-retained abutments and temporary cylinders.
The temporary cylinders were then picked up via self-cure polymethyl methacrylate. In this case, the use of digital technology to fully digitize the patient was key to achieving a predictable result functionally and esthetically (Figure 6). With its advantageous waste reduction, complex production, and cost effectiveness, 3D printing was essential to making the involved design a reality.
Conclusion
Digital dentistry is rapidly transforming the dental industry. It offers new ways to diagnose, treat, and restore teeth. Namely, 3D printing is one avenue into a more efficient and cost-effective dentistry. With its ability for rapid and accurate manufacturing and material economy, practitioners can enhance their practice positively. Current and future developments in this field can only improve this technology, with advancements in printing speeds, ceramic printing, and 4D printing serving as examples of such positive direction.
References
- Rekow E. Digital dentistry: The new state of the art — is it disruptive or destructive? Dent Mat. 2019;36:9-24.
- Miyazaki T, Hotta Y. C/D/CAM systems available for the fabrication of crown and bridge restorations. Aust Dent J. 2011;56(Suppl 1):97-106.
- Dawood A, Marti B, Sauret-Jackson V, et al. 3D printing in dentistry. Br Dent J. 2015;219:521-529.
- Giordano R. Materials for chairside CAD/CAM-produced restorationsJ J Am Dent Assoc. 2006;137:14S-21S
- Hull CW. Apparatus for production of Three-dimensional objects by stereolithography. Available at: h/tps://patents.google.com/patent/US4575330A/en. Accessed June 10, 2024.
- Celik HK, Koc S, Kustarci A, Caglayan N, Rennie AEW. The state of additive manufacturing in dental research — a systematic scoping review of 2012-2022. Heliyon. 2023;9:e17462.
- Gibson I, Rosen D, Stucker B. Additive Manufacturing Technologies: 3D Printing, Rapid Prototyping, and Direct Digital Manufacturing. 3rd ed. New York: Springer; 2015:10-13,83-86, 113
- Etemad-Shahidi Y, Qallandar OB, Evenden J, Alifui-Segbaya F, Ahmed KE. Accuracy of 3-dimensionally printed full-arch dental models: a systematic J view. J Clin Med. 2020;9:3357.
- Oberoi G, Nitsch S, Edelmayer M, Janjić K, Müller AS, Agis H. 3D printing-encompassing the facets of dentistry. Front Bioeng Biotechnol. 2018;6:172.
- Della Bona A, Cantelli V, Britto VT, Collares KF, Stansbury JW. 3D printing restorative materials using a stereolithographic technique: a systematic review. Dent Mater. 2021;37:336-350.
- Kessler A, Hickel R, Reymus M. 3D printing in dentistry-state of the art. Oper Dent. 2020;45:30-40.
- Lerner H, Nagy K, Pranno N, Zarone F, Admakin O, Mangano F. Trueness and precision of 3D-printed versus milled monolithic zirconia crowns: An in vitro study. J Dent. 2021;113:103792.
- Schweiger J, Edelhoff D, Güth JF. 3D printing in digital prosthetic dentistry: an overview of recent developments in additive manufacturing. J Clin Med. 2021;10:2010.
- Frąckiewicz W, Szymlet P, Jedliński M, Światłowska-Bajzert M, Sobolewska E. Mechanical characteristics of zirconia produced additively by 3D printing in dentistry — a systematic review with meta-analysis of novel reports. Dent Mater. 2024;40:124-138.
- Javaid M, Haleem A, Singh RP, Rab S, Suman R, Kumar L. Significance of 4D printing for dentistry: Materials, process, and potentials. J Oral Biol Craniofac Res. 2022;12:388-395.
- Celik HK, Koc S, Kustarci A, Caglayan N, Rennie AEW. The state of additive manufacturing in dental research — a systematic scoping review of 2012-2022. Heliyon. 2023;9(6):e17462.
From Decisions in Dentistry. June/July 2024; 10(4):14-16, 18