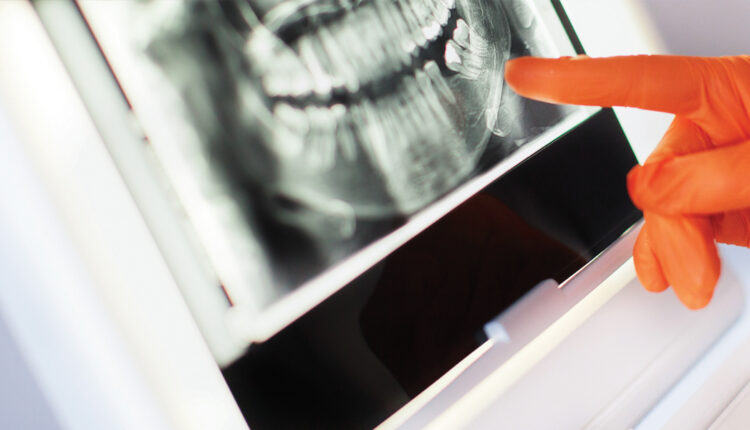
Alveolar Bone and Implant Therapy
An examination and practical application of knowledge for clinicians who place and restore dental implants.
Understanding the unique qualities of bone is important, because it facilitates anticipating healing events, helps stage therapies, and provides insight with respect to modifying osteotomy drilling protocols to accommodate various bone densities. Bone is a living tissue that protects vital organs, provides an environment for marrow, stores minerals, and supports teeth and dental implants.1 Humans are born with 300 bones and many fuse, resulting in adults having 206 distinct osseous structures.1,2 Two facial bones of special interest to dental practitioners are the maxilla and mandible. Addressing a variety of facts pertaining to alveolar bone histology, physiology and functionality, this article seeks to explain biologic events related to bone that occur prior to, during, and after dental implant placement.
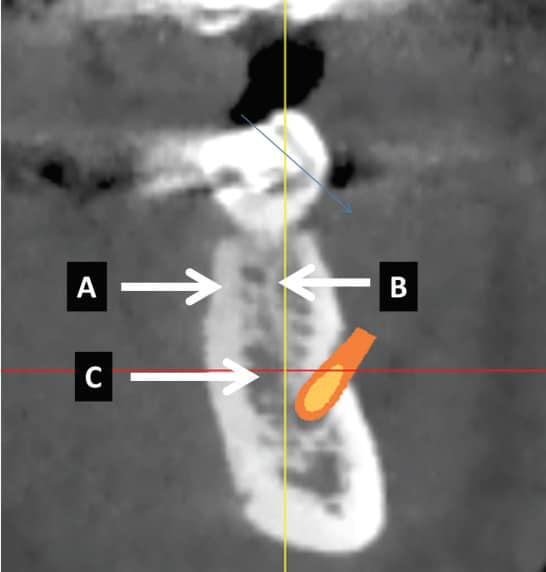
Basal bone is native bone and is present before formation of the alveolar process.3 Teeth are encased in alveolar bone, which rests upon basal bone. Histologically, these types of bones cannot be differentiated. Dental implants are usually placed into alveolar bone, and in resorbed mandibles into basal bone, whereas zygomatic implants are inserted into both types of bone.4
Bone is a specialized connective tissue that differs from other connective tissues in rigidity and hardness. Its firmness is due to inorganic salts impregnating the matrix, which consists of collagen fibers and noncollagenous proteins.5 Bone is classified as cortical (having a compact outer hard shell), or trabecular (having an inner porous network). Cortical and trabecular bone (cancellous, medullary bone) are different regarding the percentage of porosities and unit structure that comprise their macroarchitecture (Figure 1, page 28). Cortical bone has a low percentage of porosities (5% to 10%) and is considered compact, dense bone.6 Cortical bone varies in thickness from 1 to 5 mm. Its basic structural unit is the osteon (Haversian system). Osteons are typically several millimeters long and 0.2 mm in diameter, and consist of concentric lamellae of bone surrounding a central blood vessel.5 When creating an osteotomy, blood vessels in cortical bone are severed and bleeding can occur. Superficial bleeding can be managed with pressure, epinephrine from local anesthetic or cautery; hemorrhaging deep within the osteotomy is controlled by inserting an implant.
Trabecular bone has a high porosity level (75% to 95%) and can be described as a honeycombed network of enclosed spaces that contain bone marrow.5 The blood supply in trabecular bone surrounds the bone, and the blood vessels are actually in the marrow.5 The bone matrix consists of trabeculae (rods or plates), each about 200 microns (µm) thick and 1000 µm long, with marrow spaces ranging from 200 to 2000 µm.5 Large marrow spaces (e.g., 2 mm) may be found in the maxilla or mandible (Figure 1). When encountered during drilling an osteotomy over the inferior alveolar canal, it may give a false impression the inferior alveolar canal has been penetrated. A drill stop helps ensure a precise drilling depth in the presence of a large marrow space.7
In general, bone density can be classified as soft, medium or hard with respect to drill resistance when engaging cortical and cancellous bone with a 2-mm twist drill.8 Modifications of drilling protocols concerning bone density have been outlined.8 For example, when drilling an osteotomy with a 2-mm twist drill, if the bone does not feel dense, the osteotomy should be undersized so that frictional retention helps achieve primary stability (Figure 2, page 28).8 In contrast, if the bone is very dense, it is necessary to closely approximate the last drill with the implant’s diameter and use copious irrigation and sharp drills to avoid burning bone when creating an osteotomy.
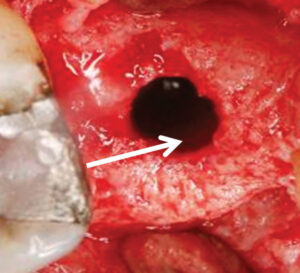
Bone has an organic (35% of weight) and inorganic (65% of weight) matrix.5 The inorganic phase is mostly hydroxyapatite, and the organic portion mainly consists of type I collagen and ground substance. It is the organic phase that allows bony plates to expand when teeth are extracted. Regarding bone mass, the cortical bone constitutes around 80% and trabecular bone provides 20%.6 However, the trabecular bone surface area is 10 times greater than the cortical bone, and this facilitates more rapid turnover and vascularization of cancellous bone.6
The main cells found within bone are osteoblasts, osteoclasts and osteocytes.5 Osteoblasts are mononucleated bone-forming cells, osteoclasts (multinucleated) resorb bone, and osteocytes are the most common bone cell and become encased in bone matrix. Osteocytes reside in lacunae and communicate with other cells via dendritic processes that traverse the bone in canaliculi (channels). They perform as mechanosensors, sense physical alterations to the bone and convey signals to adjacent cells (e.g., osteoblasts).9 Note that mechanosensors sense strain (deformation or elongation of bone in response to stress), not stress (which refers to a force acting on an object).9
In a healthy dentition with no bone or clinical attachment loss due to disease or trauma, the underlying alveolar crest follows the scallop of the cementoenamel junction (CEJ) and is approximately 2 mm apical to the CEJ.10 In the maxillary anterior region, interdental crests are around 3 mm coronal to the facial bone height (ranging from 2.1 to 4 mm).11 When placing an implant, especially in the maxillary anteriors, the implant platform is usually inserted to the level of the osseous crest on the buccal; therefore, it is common that the proximal implant surfaces are subcrestal.
SOCKET HEALING
After a tooth is removed, a blood clot forms and granulation tissue is present in the socket within 96 hours.12 Granulation tissue is transformed to connective tissue within seven to 21 days.12 Osteoid appears after 21 days, and this type of connective tissue becomes mineralized and is converted to bone.12 Woven bone (immature bone) is present in the socket in approximately three months.13 An implant can usually be inserted into woven bone (after three months) that has developed within a four-walled socket. Prior to implant placement, it is judicious to sound the bone with an anesthetic needle to determine osseous hardness. If the bone is penetrable, wait a few more months for additional mineralization to occur and sound the bone again. Bone continues to mineralize for 12 months (Figures 3A through 3D).14 Routinely, lamellar bone is present after four months.13 This is mature bone with Haversian systems.
When a socket wall is being regenerated, it is advisable to wait four to six months after bone grafting to permit bone mineralization prior to placing an implant. Similarly, when a transcrestal sinus floor elevation is performed, it is prudent to allow four to six months for bone graft mineralization prior to implant placement (Figure 3D).15 When a lateral window sinus graft is executed, bone mineralization occurs at a rate of about 1 mm per month.16 The rate of mineralization depends on how many bony walls are present adjacent to the graft material, how close the bone graft is to the bony walls, and how much bone was added. Maturation of sinus bone grafts usually takes six to nine months.
GUIDED BONE REGENERATION
Guided bone regeneration (GBR) is often performed to create bone at sites that do not have enough bone to support an implant. The principles of GBR include the following: (1) exclude epithelium and connective tissue with a barrier to permit osseous progenitor cells to repopulate the treated site; (2) create a space under the barrier, which fills with bone — the barrier is often supported with a bone graft or tenting screws or titanium reinforced barrier; (3) the barrier protects the clot; (4) achieve angiogenesis; and (5) attain primary closure by flap advancement.17 It should be noted that numerous articles have discussed performing GBR using nonresorbable dense polytetrafluorethylene barriers without primary closure to regenerate sockets that are missing an osseous wall (Figures 4A and 4B, page 30).18–20 However, the latter procedure has not been used for major horizontal or vertical alveolar ridge augmentations.20
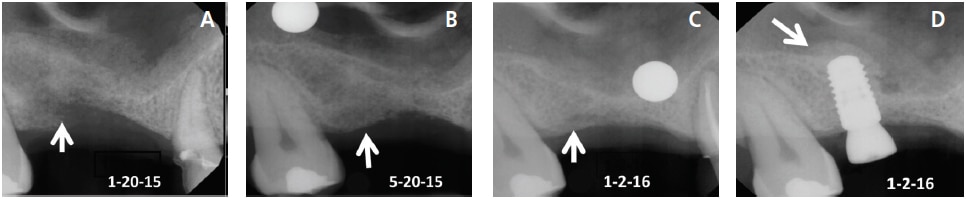
BONE HEALING AROUND A DENTAL IMPLANT
The rotational frictional force used to insert an implant into bone is called dental insertion torque.21 It affects mechanical stabilization of the implant (primary stability) and aids osseointegration.22 Primary stability indicates there is a lack of implant micromotion seen with the naked eye. In this regard, implant displacement of > 100 to 150 µm is harmful to implant osseointegration23,24 because it causes peri-implant bone remodeling, fibro-encapsulation and implant loss.23 Therefore, selecting an insertion force to provide implant stability is a critical factor for attaining predictable osseointegration.
Healing around an implant can be divided into the early and late phases.25 Upon implant placement, there is a response to a foreign material, namely, protein adsorption, platelet activation, clotting and inflammation.25 Early healing produces immature woven bone that fills the gap between the implant and bone via contact and distance osteogenesis.26 Late stages of healing include conversion of woven into lamellar bone; the remodeling of bone continues for the life of the implant.25
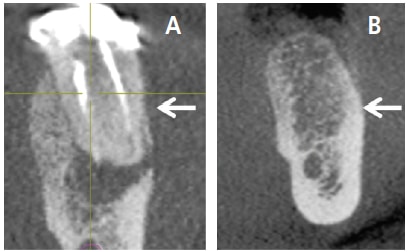
PROLIFERATIVE RESPONSE TO STRESS
Bone can react in different ways to increased mechanical load. When strain rises above a certain threshold, bone loss or deosseointegration can occur.27,28 If there is bone disuse (e.g., patient immobility), bone atrophy can result.29 In contrast, when the functional load is beneath a detrimental threshold, it can be stimulatory and prompt bone apposition and enhanced osseous density.9,30–33 These findings and other reports of bone alterations post implant insertion support the concept that bone can respond to stress and modify itself to withstand increased mechanical forces.9 The general concept that form follows function is referred to as Wollf’s law.29 With regard to osseous shape, elevated strain levels mostly affect bone modeling adjacent to the medullary cavity, thus leaving the external contour of the bone unchanged.29 This bone adaptation is believed to account for the success of prostheses associated with increased stress, such as cantilevers, increased crown-to-implant ratios, angulated abutments and teeth connected to implants.9
RATE OF BONE TURNOVER: NORMAL REMODELING
Bone remodeling provides several benefits: It removes microdamaged bone and adapts microarchitecture to strain.34 Approximately, 20% of cortical and cancellous bone surfaces (endosteal and periosteal) are remodeling at any point in time.35 Nevertheless, there are dissimilar rates of bone turnover in different bony regions; for example, alveolar bone remodels more quickly than most bones.36–38 This is possibly due to the amount of stimulation caused by occlusal function. The maxilla turns over more rapidly than the mandible,39 and cancellous bone remodels more quickly than cortical bone.40 This is due to the higher surface-to-volume ratio in cancellous bone and increased vascularity.40
The turnover rate of cortical bone is 7.7% per year and the replacement of cancellous bone is 17.7%.41 Others noted that 3% of cortical bone was changed per year and 24% of cancellous bone was replaced.39 It appears that researchers can identify a trend, but cannot delineate precisely how much bone is being remodeled yearly.
MECHANISMS OF REMODELING
There are distinct differences with respect to how cortical and trabecular bones remodel. In both types of bone, resorption and bone formation are coupled and carried out by bone multicellular units that are composed of osteoclasts and osteoblasts. In cortical bone, around 10 osteoclasts create a cutting cone (circular canal which is 2000 µm long and 150 to 200 µm wide).42 Bone is resorbed around 20 to 40 µm/day.42 Next, several thousand osteoblasts in the canal generate a secondary osteon.37 In contrast, remodeling of trabecular bone is a surface phenomenon and can occur up to 10 times faster than in cortical bone.43 Osteoclasts advance across the trabecular surface and create a trench approximately 40 to 60 µm deep, and osteoblasts produce bone.44 This helps explain why trabecular bone remodels faster than cortical bone. In addition, because drilling an osteotomy results in death of bone cells that surrounds a newly inserted implant, the remodeling described above needs to occur to replace damaged bone. This is why an implant is weakest two to four weeks after placement. Thus, procedures such as changing a healing abutment should be handled carefully or avoided during this period.
MODELING VERSUS REMODELING
There are differences that distinguish bone modeling from remodeling.45,46 Modeling alters the volumetric size of the bone (i.e., a net gain or loss), whereas remodeling does not alter bone size (Figure 5A and 5B, page 30). When tissue is modeled, there is no temporal relationship between resorption and formation of bone, while in remodeling these processes are coupled. Remodeling only affects a small percentage of bone at a location, while modeling can affect a large percentage of the bone’s surface. Remodeling is an episodic process, whereas modeling may be continuous until it is completed. During growth, the rate of modeling is greater than remodeling. However, after skeletal maturity, the rate of modeling is reduced — although remodeling continues throughout life.
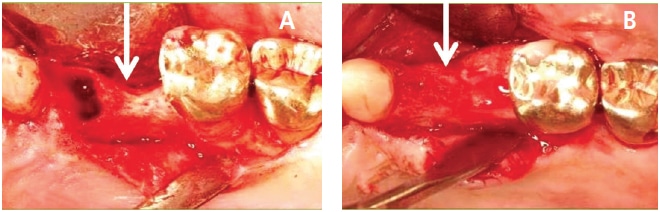
BONE LOSS RELATED TO IMPLANT PREPARATION, FUNCTIONING AND PERI-IMPLANT DISEASE
Relationship Between Blood Supply Interruption and Bone Loss: Blood is supplied to the bone around teeth via three routes: the periodontal ligament (PDL), periosteal and endosteal vasculature.47 When a tooth is extracted, PDL blood supply is disturbed, and if a flap is elevated, the periosteal blood source is further interrupted.48 Thus, if a tooth is removed and a flap is not elevated, there is reduced bone loss due to reduced interference with blood supply to the bone. A systematic review by Tan et al49 noted that if a flap is raised, the mean vertical bone and horizontal bone loss are 1.24 mm and 3.39 mm, respectively. In contrast, without flap elevation, the mean vertical bone decrease is 1 mm and the horizontal bone width decreases 0.9 to 1.2 mm.50 Accordingly, it is preferred not to elevate a buccal flap to extract a tooth, especially in the esthetic zone, to reduce bone loss and recession.
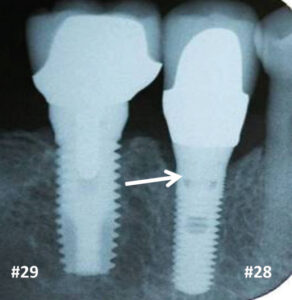
Bone Damage Due to Osteotomy Preparation and Implant Insertion: In general, it is recommended that after implant placement there should be at least 1 mm of bone surrounding the fixture to prevent post insertion bone loss.51 There is limited data addressing this topic. In this regard, Spray et al52 noted that when implants are placed into an intact ridge, if there is less than 2 mm circumferential bone, vertical bone loss occurs after implant placement. However, these data were collected when flap procedures were performed. It is unclear how much bone needs to be present to inhibit bone loss if flapless procedures are used to place implants. Concerning immediate implant placement, it is usually recommended that implants be inserted 1 mm subcrestally with respect to the buccal plate to accommodate the 1 mm of vertical bone loss that commonly occurs after extractions.49,50,53 To reduce osseous resorption when doing an immediate implant, the following steps should be taken: no flap elevation, atraumatic extraction, bone graft placed into the buccal gap, and retention of this bone with a healing abutment or a provisional prosthesis.54,55
Microcracks may be created when an osteotomy is developed or when a dental implant is inserted.56 A microcrack is a fissure or break in the hydroxyapatite,57 and remodeling repairs these defects.58 However, microcracks subjected to prolonged loading can develop macrocracks, which can cause bone fracture and bone deterioration.59,60
Historically, animal investigations indicated that bone is resorbed during the first year, and this was attributed to biologic width formation.61,62 Investigators noted that if implants are submerged, bone loss does not occur until an abutment is attached and a microgap is created.61 In humans, two-piece implants that were originally submerged were found to lose 1 to 1.5 mm of vertical bone height by the end of the first year (Figure 6).63–65 In contrast, one-piece implants (i.e., tissue-level implants) whose platforms were positioned supracrestally lost 0.75 to 1 mm of bone during the first year.66–68 The reduced amount of bone resorption is due to supracrestal biologic width formation (Figure 6). Note, the above data refers to non-platform-switched abutments. In contrast, Atieh et al69 reported that platform switching (i.e., the abutment is narrower than the platform), resulted in less bone loss around implants than those restored without platform switching — means 0.055 to 0.99 mm versus 0.19 to 1.67 mm (Figure 7).
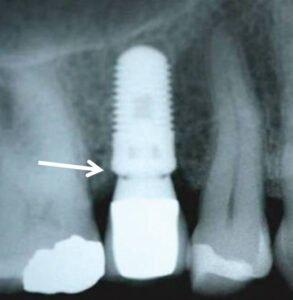
Bone Loss due to Positioning of Dental Implants: To avoid losing bone between two non-platform-switched implants, their distance apart should be 3 mm.70 If the distance is < 3 mm, vertical osseous resorption is 1.04 mm. When the inter implant distance is > 3mm, a mean of about 0.45 mm vertical bone loss occurs. The space between an implant and a tooth ought to be 1.5 to 2 mm.71 Esposito et al71 reported that bone loss was inversely proportional to how close the implant is to the tooth. In general, if implants are inserted too close to each other or an adjacent tooth, it can result in excessive inter-implant bone resorption, loss of papilla, increased recession, poor esthetics, incorrect crown contours and food retention. Note, platform-switched implants can be placed a little closer together without increasing the amount of bone loss.72
Peri-implantitis: This is a destructive inflammatory process affecting soft and hard tissues surrounding dental implants, and numerous articles have addressed the subject.73 The results of a 2013 systematic review indicated that 18.8% of patients (9.6% of the implants) develop peri-implantitis.74 Accordingly, maintenance and periodic monitoring of clinical attachment or bone levels around implants is important because it facilitates early detection of peri-implantitis. Timely intervention is essential to prevent continued disease progression, which can result in implant failure.
Bone Loss Associated Occlusal Overload: There are studies that suggest occlusal overload causes deosseointegration27 or progressive bone loss,75 or has no effect;76 thus, the information in the literature is conflicting. In this regard, a recent review assessed animal and human studies and concluded that no decisive conclusion can be drawn with respect to the effect of occlusal overload on bone loss.77 Another systematic review was more definitive and concluded occlusal overload on uninflamed peri-implant bone tissue did not cause bone breakdown, whereas if it was combined with inflammation, it increased plaque-induced bone resorption.78 Nevertheless, there are situations in which bone loss occurs in the absence of clinical inflammation, and it has been attributed to occlusal trauma.75
CONCLUSION
This discussion of bone correlates basic science to events that occur when treating patient who have been restored with dental implants. Familiarity with data pertaining to bone histology, physiology, bone types, and stages of healing affects treatment planning and how providers approach implant therapy.
KEY TAKEAWAYS
- An understanding of biologic events related to alveolar bone histology, physiology and functionality will help support successful outcomes in implant therapy.
- Dental implants are usually placed into alveolar bone, and in resorbed mandibles into basal bone, whereas zygomatic implants are inserted into both types of bone.4
- An implant can usually be inserted into woven bone (after three months) that has developed within a four-walled socket.
- Prior to implant placement, it is judicious to determine osseous hardness by sounding the bone with an anesthetic needle.
- Guided bone regeneration is often performed to create bone at sites that do not have enough bone to support a dental implant.
- In general, it is recommended that after implant placement there should be at least 1 mm of bone surrounding the fixture to prevent post insertion bone loss.51
References
- International Osteoporosis Foundation. Introduction to Bone Biology: All About our Bones. Available at: iofbonehealth.org/introduction-bone-biology-all-about-our-bones. Accessed April 10, 2018.
- Norton NS. Osteology. In: Netter’s Head and Neck Anatomy for Dentistry. Philadelphia: Saunders; 2007: 25-65.
- Park HS, Lee YJ, Jeong SH, Kwon TG. Density of the alveolar and basal bones of the maxilla and the mandible. Am J Orthod Dentofacial Orthop 2008;133:30–37.
- Chrcanovic BR, Albrektsson T, Wennerberg A. Survival and complications of zygomatic implants: an updated systematic review. J Oral Maxillofac Surg. 2016;74:1949–1964.
- BME/ME 456 Biomechanics. Bone Structure. Available at: http://mujweb.cz/zemanici/Petra%20skola/Studium%20PGS/internet%20klinicka%20biomechanika/HTML/bonestructure.htm. Accessed April 19, 2018.
- University of Michigan. BME 332: Introduction to Biosolid Mechanics. Available at: umich.edu/~bme332/ch0overview/bme332overview.htm. Accessed April 19, 2018.
- Greenstein G. Greenstein B, Desai R. Using drill stops on twist drills to promote safety and efficiency when creating osteotomies for dental implants. J Am Dent Assoc. 2014;145:371–375.
- Cavallaro J, Greenstein B, Greenstein G. Clinical methodologies for achieving primary dental implant stability. J Am Dent Assoc. 2009:140:1366–1372.
- Greenstein G, Cavallaro J, Tarnow D. Assessing bone’s adaptive capacity around dental implants. J Am Dent Assoc. 2013;144:362–368.
- Ercan E, Celikoglu M, Buyuk SK, Sekerci AE. Assessment of the alveolar bone support of patients with unilateral cleft lip and palate: a cone-beam computed tomography study. Angle Orthod. 2015;85:1003–1008.
- Becker W, Ochsenbein C, Tibbets L, Becker BE. Alveolar bone anatomic profiles as measured from dry skulls. J Clin Periodontol. 1997;24:727–731.
- Amler MH, Johnson Pl, Salman I. Histological and histochemical investigation of human alveolar socket healing in undisturbed extraction wounds. J Am Dent Assoc. 1960;61:32–44.
- Eriksson C, Ohlson K, Richter K, Billerdahl N, Johansson M, Nygren H. Callus formation and remodeling at titanium implants. J Biomed Mater Res A. 2007;83:1062–1069.
- Minichetti JC, D’Amore JC, Hong AY, Cleveland DB. Human histologic analysis of mineralized bone allograft (Puros) placement before implant surgery. J Oral Implantol. 2004;30:74–82.
- Greenstein G, Cavallaro J. Transcrestal sinus floor elevation with osteotomes: simplified technique for medium dense bone and management of various scenarios. Compend Contin Educ Dent. 2011; 32:14–20.
- Davies JE, Hosseini MM. Histodynamics of endosseous wound healing. Bone Engineering. 2001;1:1–13.
- Bashutski J, Oh TJ, Chan HL, Wang HL. Guided tissue regeneration: a decision-making model. J Int Acad Periodontol. 2011;13:48–57.
- Hoffman O, Bartee BK, Beaumont C, Kasaj A, Deli G, Zafiropoulos GG. Alveolar bone preservation in extraction sockets using non-resorbable dPTFE membranes: a retrospective non-randomized study. J Periodontol. 2008;79:1355–1369.
- Carbonell JM, Martín IS, Santos A, Pujol A, Sanz-Moliner JD, Nart J. High-density polytetrafluoroethylene membranes in guided bone and tissue regeneration procedures: a literature review. Int J Oral Maxillofac Surg. 2014;43:75–84.
- Greenstein G, Carpentieri JR. Utilization of d-PTFE barriers for post-extraction bone regeneration in preparation for dental implants. Compend Contin Educ Dent. 2015;36:465–473.
- 21.Trisi P, Todisco M, Consolo U, Travaglini D. High versus low implant insertion torque: a histologic, histomorphometric, and biomechanical study in the sheep mandible. Int J Oral Maxillofac Implants. 2011; 26:837–849.
- Trisi P, De Benedittis S, Perfetti G, Berardi D. Primary stability, insertion torque and bone density of cylindric implant ad modum Branemark: is there a relationship? An in vitro study. Clin Oral Implants Res. 2011;22:567–570.
- Szmukler-Moncler S, Piattelli A, Favero GA, Dubruille JH. Considerations preliminary to the application of early and immediate loading protocols in dental implantology. Clin Oral Implants Res. 2000;11:12–25.
- Pilliar RM, Lee JM, Maniatopoulos C. Observations on the effect of movement on bone in growth into porous-surfaced implants. Clin Orthop Relat Res. 1986;208:108–313.
- Kuzyk PR, Schemitsch EH. The basic science of peri-implant bone healing. Indian J Orthop. 2011;45:108–115.
- Davies JE. Understanding peri-implant endosseous healing. J Dent Educ. 2003;67:932–949.
- Isidor F. Loss of osseointegration caused by occlusal load of oral implants: a clinical and radiographic study in monkeys. Clin Oral Implants Res. 1996;7:143–152.
- Chambrone L, Chambrone LA, Lima LA. Effects of occlusal overload on peri-implant tissue health: a systematic review of animal-model studies. J Periodontol. 2010;81:1367–1378.
- Ruff C, Holt B, Trinkaus E. Who’s afraid of the big bad Wolff?: “Wolff’s law” and bone functional adaptation. Am J Phys Anthropol. 2006;129:484–498.
- Gotfredsen K, Berglundh T, Lindhe J. Bone reactions adjacent to titanium implants subjected to static load of different duration. A study in the dog (III). Clin Oral Implants Res. 2001;12:552–558.
- 31 Yunus B. Assessment of the increased calcification of the jaw bone with CT-Scan after dental implant placement. Imaging Sci Dent. 2011;41:59–62.
- Bass SL, Saxon L, Daly RM, et al. The effect of mechanical loading on the size and shape of bone in pre-, peri-, and postpubertal girls: a study in tennis players. J Bone Miner Res. 2002;17:2274–2280.
- Garat JA, Gordillo ME, Ubios AM. Bone response to different strength orthodontic forces in animals with periodontitis. J Periodontal Res. 2005;40:441–445.
- Lee CS, Szczesny SE, Soslowsky LJ. Remodeling and repair of orthopedic tissue: role of mechanical loading and biologics: part II: cartilage and bone. Am J Orthop. 2011;40:122–128.
- Jee WSS. Integrated Bone Tissue Physiology: Anatomy and Physiology. In: Cowin SC, ed. Bone Mechanics Handbook. 2nd ed. Boca Raton, Florida: CRC Press; 2001:1–28.
- Garetto LP, Chen J, Parr JA, Roberts WE. Remodeling dynamics of bone supporting rigidly fixed titanium implants: a histomorphometric comparison in four species including humans. Implant Dent. 1995;4:235–243.
- Tricker ND, Dixon RB, Garetto LP. Cortical bone turnover and mineral apposition in dentate bone mandible. In: Garetto LP, Turner CH, Duncan RL, Burr DB, eds. Bridging the gap between dental and orthopaedic implants. Indianapolis, Indiana: School of Dentistry Indiana University; 2002:226–227.
- Huja SS, Fernandez SA, Hill KJ, Li Y. Remodeling dynamics in the alveolar process in skeletally mature dogs. Anat Rec A Discov Mol Cell Evol Biol. 2006;288:1243–1249.
- Deguchi T, Takano-Yamamoto T, Yabuuchi T, Ando R, Roberts WE, Garetto LP. Histomorphometric evaluation of alveolar bone turnover between the maxilla and the mandible during experimental tooth movement in dogs. Am J Orthod Dentofacial Orthop. 2008;133:889–897.
- Parfitt AM. Bone remodeling. Henry Ford Hosp Med J. 1988;36:143–144.
- Gurnero P, Szulic DA. Bone remodeling: cellular activities in bone. In: Orwoll E, Bilezikian JP, Vanderschueren D, eds. Osteoporosis in men: the effects of gender on skeletal health. 2nd ed. London, United Kingdom: Elsevier; 2010;15–24.
- Petrtýl M, Hert J, Fiala P. Spatial organization of the haversian bone in man. J Biomech. 1996;29:161–169.
- Lee CA, Einhorn TA. The bone organ system. In: Marcus R, Feldman D, Kelsey J, eds. Osteoporosis. 2nd ed. Academic Press, San Diego; 2001:3–20.
- Mosekilde L. Consequences of the remodeling process for vertebral trabecular bone structure: a scanning electron microscopy study (uncoupling of unloaded structures). Bone Miner. 1990;10:13–35.
- Seeman E. Bone modeling and remodeling. Crit Rev Eukaryot Gene Expr. 2009;19:219–233.
- Randall LE. Bone Remodeling Surrounding Primary Teeth in the Maxilla and Mandible of Skeletally Immature Dogs. Thesis. The Ohio State University 2010. Available at: https://etd.ohiolink.edu/rws_etd/document/get/osu1268087680/inline. Accessed April 19, 2018.
- Al-Sabbagh M. Complications in implant dentistry. Dent Clin North Am. 2015;59:8–15.
- Elian N, Cho SC, Froum S, Smith RB, Tarnow DP. A simplified socket classification and repair technique. Pract Proced Aesthet Dent. 2007;19:99–104.
- Tan WL, Wong TL, Wong MC, Lang NP. A systematic review of post-extraction alveolar hard and soft tissue dimensional changes in humans. Clin Oral Implants Res. 2012;23(Suppl 5):1–21.
- Lee CT, Chiu TS, Chuang SK, Tarnow D, Stoupel J. Alterations of the bone dimension following immediate implant placement into extraction socket: systematic review and meta-analysis. J Clin Periodontol. 2014;41:914–926.
- Belser UC, Bernard JP, Buser D. Implants in the esthetic zone. In: Lindhe J, Lang NP, Karring T, eds. 5th ed. Clinical Periodontology and Implant Dentistry. Oxford: Blackwell Publishing Ltd; 2008:1146–1174.
- Spray JR, Black CG, Morris HF, Ochi S. The influence of bone thickness on facial marginal bone response: stage 1 placement through stage 2 uncovering. Ann Periodontol. 2000;5:119–128.
- Araújo MG, Lindhe J. Dimensional ridge alterations following tooth extraction. An experimental study in the dog. J Clin Periodontol. 2005;32:212–218.
- Mehta H, Shah S. Management of buccal gap and resorption of buccal plate in immediate implant placement: a clinical case report. J Int Oral Health. 2015;7(Suppl 1):72–75.
- Tarnow DP, Chu SJ, Salama MA, et al. Flapless postextraction socket implant placement in the esthetic zone: part 1. The effect of bone grafting and/or provisional restoration on facial-palatal ridge dimensional change-a retrospective cohort study. Int J Periodontics Restorative Dent. 2014;34:323–331.
- Huja SS, Katona TR, Burr DB, Garetto LP, Roberts WE. Microdamage adjacent to endosseous implants. Bone. 1999;25:217–222.
- Lee TC, Mohsin S, Taylor D, et al. Detecting microdamage in bone. J Anat. 2003;203:161–172.
- Warreth A, Polyzois I, Lee CT, Claffey N. Generation of microdamage around endosseous implants. Clin Oral Implants Res. 2009;20:1300–1306.
- Donahue SW, Galley SA. Microdamage in bone: implications for fracture, repair, remodeling, and adaptation. Crit Rev Biomed Eng. 2006;34:215–271.
- O’Brien FJ, Hardiman DA, Hazenberg JG, et al. The behaviour of microcracks in compact bone. Eur J Morphol. 2005;42:71–79.
- Hermann JS, Cochran DL, Nummikoski PV, Buser D. Crestal bone changes around titanium implants. A radiographic evaluation of unloaded nonsubmerged and submerged implants in the canine mandible. J Periodontol. 1997;68:1117–1130.
- Hermann JS, Schoolfield JD, Nummikoski PV, Buser D, Schenk RK, Cochran DL. Crestal bone changes around titanium implants: a methodologic study comparing linear radiographic with histometric measurements. Int J Oral Maxillofac Implants. 2001;16:475–485.
- Hartman GA, Cochran DL. Initial implant position determines the magnitude of crestal bone remodeling. J Periodontol. 2004;75:572–577.
- Bryant SR, Zarb GA. Crestal bone loss proximal to oral implants in older and younger adults. J Prosthet Dent. 2003;89:589–597.
- Smith DE, Zarb GA. Criteria for success of osseointegrated endosseous implants. J Prosthet Dent. 1989;62:567–572.
- Weber HP, Crohin CC, Fiorellini JP. A 5-year prospective clinical and radiographic study of nonsubmerged dental implants. Clin Oral Implant Res. 2000;11:144–153.
- Weber HP, Buser D, Fiorellini JP, Williams RC. Radiographic evaluation of crestal bone levels adjacent to nonsubmerged titanium implants. Clin Oral Impl Res. 1992;3:181–188.
- Brägger U, Häfeli U, Huber B, Hämmerle CH, Lang NP. Evaluation of postsurgical crestal bone levels adjacent to nonsubmerged dental implants. Clin Oral Impl Res. 1998;9:218–224.
- Atieh MA, Ibrahim HM, Atieh AH. Platform switching for marginal bone preservation around dental implants: a systematic review and meta-analysis.
J Periodontol. 2010;81:1350–1366. - Tarnow DP, Cho SC, Wallace SS. The effect of inter-implant distance on the height of inter-implant bone crest. J Periodontol. 2000;71:546–549.
- Esposito M, Ekestubbe A, Gröndahl K. Radiological evaluation of marginal bone loss at tooth surfaces facing single Brånemark implants. Clin Oral Implants Res. 1993;4:151–157.
- Rodríguez-Ciurana X, Vela-Nebot X, Segalà-Torres M, et al. The effect of interimplant distance on the height of the interimplant bone crest when using platform-switched implants. Int J Periodontics Restorative Dent. 2009;29:141–151.
- Ting M, Craig J, Balkin BE, Suzuki JB. Peri-implantitis: a comprehensive overview of systematic reviews. J Oral Implantol. 2017;28.
- Atieh MA, Alsabeeha NH, Faggion CM Jr, Duncan WJ. The frequency of peri-implant diseases: a systematic review and meta-analysis. J Periodontol. 2013;84:1586–1598.
- Misch CE, Suzuki JB, Misch-Dietsh FM, Bidez MW. A positive correlation between occlusal trauma and peri-implant bone loss: literature support. Implant Dent. 2005;14:108–116.
- Heitz-Mayfield LJ, Schmid B, Weigel C, et al. Does excessive occlusal load affect osseointegration? An experimental study in the dog. Clin Oral Implants Res. 2004;15:259–268.
- Graves CV, Harrel SK, Rossmann JA, et al. The role of occlusion in the dental implant and peri-implant condition: a review. Open Dent J. 2016;10:594–601.
- Afrashtehfar KI, Afrashtehfar CD. Lack of association between overload and peri-implant tissue loss in healthy conditions. Evid Based Dent. 2016;17:92–93.
Featured image by BRANIMIR76/E+/GETTY IMAGES PLUS
From Decisions in Dentistry. May 2018;4(5):26-32.
[…] Medal Award: Gary Greenstein, DDS, MS. Greenstein’s robust publishing portfolio has provided comprehensive insight to the intricacies […]